13.2 Introduction
Soil temperature profoundly influences many biological processes, including plant growth and development, and the behavior of soil-dwelling microorganisms, insects, and higher animals. Much of the early work on soil temperature effects on plant growth is summarized in Richards et al. (1952). As an example, consider the effects of low temperature on transpiration (Fig 13.1). The warm weather plants, cotton and watermelon, are significantly more sensitive to cold temperatures than are collards, which are grown as a winter crop in the southern United States. Plants are also sensitive to the extremes of temperature between day and night, winter and summer. In many species, it is this fluctuation of temperature rather than its absolute or average value which is the key ingredient, as for example in seed germination (see e.g., Richards et al. 1952). Consequently, a complete understanding of the soil temperature dependence of plant growth and development requires knowledge of soil temperature as a function of time. As will be pointed out later, soil temperature variation with depth is another factor of importance here. The metabolic processes of soil-dwelling microorganisms are also temperature-dependent. They break down organic matter, produce nitrogen, and play a part in the aggregation of the soil itself (Richards et al. 1952). In turn, these processes affect plant growth and maintenance of the nutrient cycle. Monteith (1973) points out, however, that it is difficult to observe the behavior of the flora and fauna in an undisturbed, natural soil. Thus relatively little is known concerning temperature effects on them. Certainly, however, a model of soil temperature variation is an essential first step on the road to this understanding.
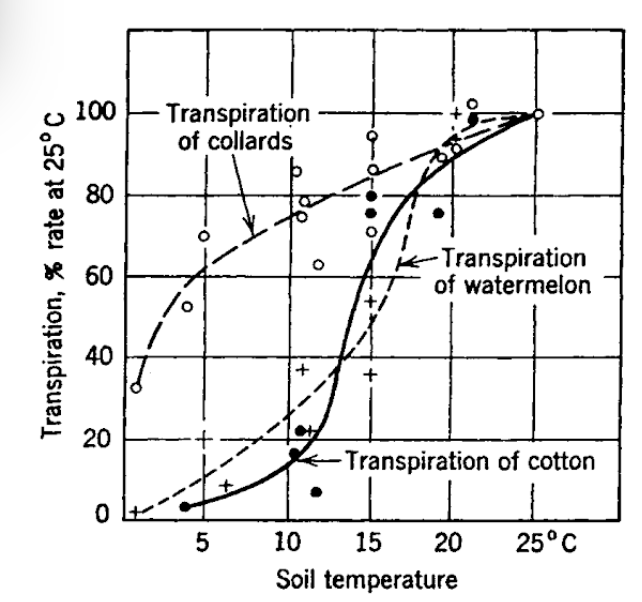
Figure 13.1: Effects of low soil temperatures on water absorption by four species of plants, as measured by rates of transpiration (from Kramer 1969).
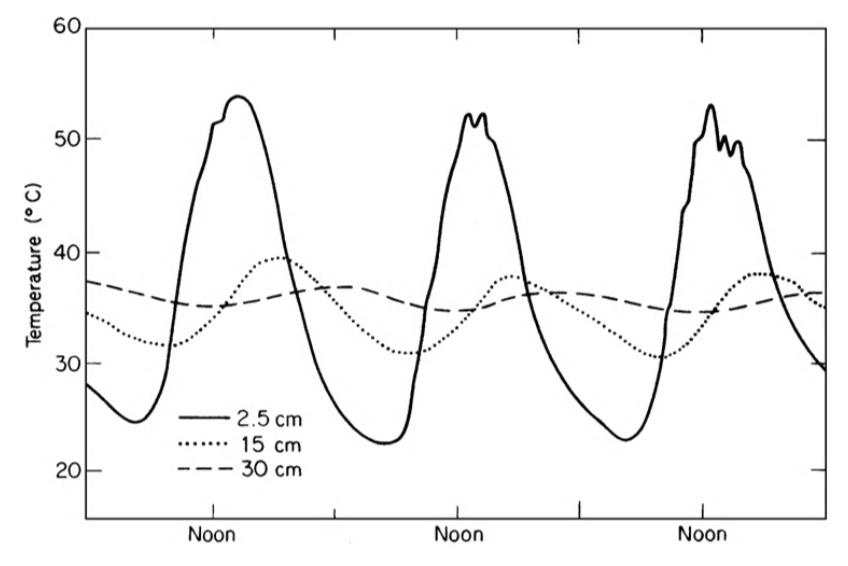
Figure 13.2: Diurnal course of temperature at three depths in a sandy loam beneath a bare uncultivated surface; Grifith, New South Wales, 17-19 January, 1939 (from Monteith 1973).
Finally, consider some higher animals who spend part of their life cycle in the soil. These include obvious soil-dwellers such as mice, gophers, moles, etc. In addition, hibernating species such as bears become closely coupled to soil temperatures during their inactive period. The animal ecologist is often concerned with elucidating how the survival strategies of such animals are related to the physical world around them; not only in qualitative terms, but also in terms of measurable parameters. As an example, consider the pocket mouse, Perognathus longimembris, which, with a body weight of ~ 8 g, is among the smallest rodents. French (1976), in a series of laboratory experiments, determined that this species selected the highest experimental temperature in the soil for its burrow at all times of the year. The question of determining the mouse’s location at any time of the day or year becomes in large part, then, a question of determining where the maximum soil temperature occurs in the field. On the other hand, some animals that hibernate prefer lower temperatures near their dormant body temperature in order to conserve energy while in the dormant state. In short, many survival strategies of animals are affected to one degree or another by the soil temperature environment.
Soil heat flow and the resulting soil temperature distributions have important ecological consequences. The question remains, however, as to how soil temperatures can be obtained as a practical matter. Two alternatives present themselves. In some applications, actual measurement of soil temperature profiles and heat flux is preferred. This approach is straightforward, assuming the proper instrumentation is available. Surface and profile heterogeneity may make extensive sampling necessary, however. Actual measurements of soil temperatures are frequently not available, whether due to the expense of the equipment, inaccessibility of the site, or simply due to the inconvenience involved. Consequently, the utility of a model which can predict soil temperatures from more readily attainable environmental variables becomes apparent.
This module will focus primarily on the modelling approach. It should be pointed out that much of the physical insight developed here is also essential for establishing a proper measurement program. In the final analysis, the type of approach utilized will depend upon individual circumstances.