10 Effective ecology
How to cite this chapter
Cousens R. D. (2023) Effective ecology. In: Cousens R. D. (ed.) Effective ecology: seeking success in a hard science. CRC Press, Taylor & Francis, Milton Park, United Kingdom, pp. 173-182. Doi:10.1201/9781003314332-10
Abstract This chapter ties together the major threads from the book: the need for clarity of goals, for critical analysis of impediments and pathways to achieve those goals and for rigour in the delivery of research and its interpretation. More effective research by the individual researcher requires changes in attitude, which will only come from an acceptance of the value of change achieved through better communication and education. The chapter also proposes changes that might be introduced into the broader network of Ecology to help it become more effective. Here, too, there are barriers to shifting the status quo of organisations and individuals. Little will change without those in leadership roles inside and outside Ecology recognising that action is needed and long overdue. How do we achieve that?
10.1 How to increase the effectiveness of ecological research
It would be great to finish with a chapter that gives instructions, a recipe or a ‘road map’ to success: follow these steps and everyone will be the ultimate, effective ecologist… But no! The many factors that make ecology difficult will persist and progress will always be hard. There are no magic recipes for success in ecology, despite the prevalence of popular research techniques in our publications! The approach required depends on each researcher’s aim in any particular instance, but it is possible to identify generally good and bad research practices, and to provide some guidance.
Problem-solving requires a knowledge of what the problems are and it requires vigilance; critique needs to become a habit. That is why the book’s authors have identified the sorts of problems that face us, and the hurdles that we must attempt to overcome, rather than providing specific solutions to the problems. Some of the hurdles may be overcome by a combination of skill, ingenuity, critical evaluation and good research design. Different researchers may identify different solutions, but many will be only partially effective. There is no perfect solution and good judgement is required to achieve even partial insight. Some barriers may be insurmountable (at least for now): we must identify these and not waste time on misguided research and misleading inferences.
A more critical approach to ecology should achieve more effective research. Stronger criticism of ourselves and of others may be the best recipe for effective ecology. By questioning everything, perhaps against our instincts to trust and respect our peers, we are more likely to identify what is holding us back. Although a piece of research may appear good and interesting, issues may become apparent upon closer scrutiny. It is not a case of trying to find faults, but of finding a better way, a way of answering a question more effectively. For example, a particular study may lack certain approaches that were beyond the skills of the researchers; this could be resolved by finding collaborators who have those skills.
As we discussed in Chapter 2, Ecology evolves as the outcome of the behaviours of the individual researchers. Therefore, we need to examine how individuals make project design decisions. For simplicity, consider design as three phases: goals (the outcomes sought); actions (our modi operandi); and application (how we proceed with actions). As discussed in Chapter 2, however, the evolution of Ecology into its current form is embedded in and constrained by the multilayer network that is Ecology and all the interactions it includes (Figure 2.2). We must therefore consider all the various layers and nodes in the network and determine how they can be modified to make the whole system more effective.
Although the authors of the book are all proud of what Ecology has achieved, and confident in its ability to deliver excellence, we are also certain that ecology can be more effective; it is not ineffective currently, but it could be better. The question is: how?
10.2 What we seek to achieve
In The role of critique in science in Chapter 1, we argued that the effectiveness of a project should be evaluated based on its goals. We did not suggest that merely having a goal will result in better research outcomes, but goal orientation in planning research makes sense. It seems logical to say ‘this is what I want to achieve, and this set of actions appears the most likely to succeed’. The alternative is to believe that following a program of habitual actions based on experience will, somehow, lead to benefits. Many ecologists have built successful careers on this latter model. Goal-orientated research does not necessarily produce outcomes that are narrower; and both approaches can lead to unexpected spin-offs. An explicit goal provides greater likelihood that the outcomes include those intended.
There are practical reasons why good intentions to be goal-driven may not reach fruition. One is funding. Resources are limited and usually competitive. Do we determine our scientific goal, work out the budget required and then try to find that funding? The resource requirement may be beyond the limits of granting agencies or the project may not fit their criteria. Unconventional (for scientists) entrepreneurial, lobbying and collaboration skills may be required to find atypical funding. The effort required may be great, but a rare success may be worth the time invested. This is how the CERN and Deep Space networks came about. It is also how we need to proceed if ecological research is to be internationally coordinated and funded to tackle the most important goals. The alternative is to start with the budget (e.g. an agency’s project funding limit) and design a project with an objective that fits the budget. The purse limits the proposal scope, but success rates can still be low. This is the way many of us play the funding game. It can provide scientifically valuable research, but probably not be what we really need.
Most ecologists have some idea of an end-point for their research, perhaps in their subconscious, but it may not be explicit, perhaps because it is rarely given the opportunity to develop. Early on in our careers our approaches to research are determined for us. Initially, we inherit (or accept) a research style from our supervisors and bosses, but over time, we gain more flexibility and respond to other influences. We are influenced most by those whose research impresses us, those who judge our work and those who pay for our research (usually the same bodies that set the criteria on which we are judged). It seems quite likely that these people will not be goal-orientated in the same way and we act accordingly and conservatively. The status quo is maintained. Under a system of passive diffusion of ideas, even the most influential goal-driven research will take a long time to change researcher behaviour.
So… How could the Ecology network and its components become more goal-orientated?
If funding agencies were to ask researchers for explicit long-term goals for their programs and not just the expectations for current projects, then the applicants and reviewers would react accordingly. Researchers would set explicit goals for peers to adjudicate (see Ecological Goals in Chapter 1). Moreover, if the agency’s priorities specified types of outcome, not just fields of activity, very different projects would be submitted. For example, rather than broad ‘research on ecological aspects of climate change’, the agency could specify mitigation options for known climate impacts. Such a shift in policy would require a clear and radical decision by a leader with authority who understood the benefits of goal-setting.
Another layer in the ecological network is our professional societies. Could these play a part in encouraging more goal-focused research? While societies fill many valuable roles, including knowledge dissemination, they have generally not played a role in setting the scientific agenda. One exception was a survey, with repeated rounds of voting and discussion, conducted by the British Ecological Society (Sutherland et al. 2013) which produced a list of 100 ‘questions of fundamental importance’, divided into seven subject areas. We can imagine a similar exercise focusing on the goals to be achieved. More recently, that same society has been undertaking a process ‘to develop a unified community vision of the grand challenges for ecology and how best these can be met’ (British Ecological Society 2022). Societies could also have influence on behaviours through themed symposia in their conferences or through plenary sessions addressed by leading exponents of goal-focused research.
Finally, and most importantly, we are influenced by active discourse with others in our field. Workshops have a long tradition in ecology, often including formal presentations and posters, but with time allocated to the exploration and synthesis of ideas. Typically, workshops produce lists of future research opportunities (i.e. things to do). Sets of goals (to achieve) and challenges to peers are less common outputs. Conventional workshops can be transformed into effective debates about goals and challenges; it just needs a different philosophy from the organisers. The Andina workshops (Cousens 2017), which were mentioned in the Preface, were set up to provide an environment for open debate, with an emphasis on bringing together early-career and more experienced researchers. They are always over-subscribed. Up to five discussion papers have been published from each of the six biennial workshops thus far and feedback from attendees has been outstanding. Another example of goal-orientated workshops is the ‘sandpits’ scheme run over five days by the Engineering and Physical Sciences Research Council in the UK (UK Research and Innovation 2021). That program uses lateral thinking and radical approaches to address research challenges, and the outcomes can include funding for projects.
A perennial problem for all debate-style workshops is funding. They lack economy of scale and have limits on attendance; to be effective, they need more than just one or two days. Each Andina meeting faces a funding challenge that must be solved. Some ecological societies provide limited funds for short meetings of members’ working groups, but their scope is often very restricted. Other workshops are ad hoc, organised by informal networks of colleagues, perhaps with funding included in a research grant (and, sadly, often by invitation only). NCEAS, one of the most successful long-term centres for ecological synthesis (and discourse: Halpern et al. 2020), received core funding from the National Science Foundation in the US from 1995 to 2010. This, and similar centres, have been forced to close or to develop alternative and often less secure funding (Baron et al. 2017). If ecologists are to take goal-setting workshops seriously, we need organisations, whether societies or government agencies, to recognise them as priorities and to allocate sufficient funds. This may divert some of the limited resources from actual research, but it may prove more effective in the long term.
10.3 How we go about ecology
Ecology is a multidimensional science: there are many things that we do and many ways we do them. There has never been a comprehensive analysis of everything that we do, only restricted discussions on particular methods or broad issues. In a survey of almost 111,000 articles in 136 journals, Carmel et al. (2013) classified research as observational (59%), experimental (28%), modelling (12%), analysis of existing data (9%) or problem-solving (17%) [note that some articles were scored in multiple categories]. Other studies have focused on the topics we research and the vocabulary we use (Andersen et al. 2021), rather than how we do the research. This section will focus on just three overlapping and common approaches to ecological research: our acceptance of weak inference from descriptive observations; research that focuses on system outputs rather than ecological processes; and the collation of evidence that supports a particular proposition.
10.3.1 Descriptive ecology
Ecology’s history began with description. This is how we generate our ideas and hypotheses: we collect data, summarise and analyse them as best we can and then speculate on explanations. Only then can we start to test our ideas.
Carmel et al. (2013) found that descriptive studies using data exploration methods still represent over half of published papers. Indeed, the ‘new era’ of data-driven ecology (McCallen et al., 2019), big data and deep learning (Guo et al. 2020) is still a form of description although the wealth of detail makes more perceptive analysis possible. Remember, however, that big ecological data sets are usually full of autocorrelation, in both space and time, and autocorrelation can strongly influence both analysis and interpretation (Dale and Fortin 2014). All that really differs from past efforts are the tools now at our disposal. Ecology has maintained an approach in which the analysis of pattern still dominates as an attempt to understand process (McCallen et al. 2019).
As Ecology matures in seeking insights more definite than speculation, efforts may swing to more investigative approaches, using experiments and modelling, but descriptive work still dominates, in part because the natural world is so diverse that there are many things not yet described. Plausible explanations are developed based on intuition or expert opinion, on comparisons with other systems (perhaps including experimental results) or from detecting relationships between variables (such as correlations). Although correlation is limited in determining causal relationships for several reasons, many ecologists are happy to leave their research at that point (see Chapter 7 for prime examples of this). The correlation gives them a plausible explanation for their observations and that is sufficient; or perhaps any further progress is beyond their technical or resource capabilities.
Predictive capacity is a valid goal and does not ipso facto require a knowledge of mechanisms (see Chapter 3) and so we cannot fault those who seek to establish it through correlation. Indeed, it can be argued that our insistence on explanations has been somewhat counter-productive (Currie 2018). Many years of research can lead to only a partial understanding of mechanisms, with a long list of caveats and little ability to predict. The search for mechanistic understanding will never be complete and will always require further work. Correlations, however, offer an ability to predict statistically even in the absence of understanding. Provided that the limitations of the approach are fully appreciated and nothing changes, we should be able to develop some robust rules and generalisations through descriptive data alone. One major concern is that correlative prediction requires stationarity (i.e. that statistical properties of data do not change over space or time). If the processes are not stationary, we need to know more than correlation, otherwise our conclusions can be spurious and unreliable.
If we want to progress from speculation about mechanisms towards better justified, confident statements about ecological processes, then we need to proceed through a sequence of steps of questions and answers (as discussed in Chapter 6 and depicted in Figures 1.1b and c). Correlations generate hypotheses, which may be followed up experimentally to put together a stronger body of evidence (regardless of whether a hypothesis can be categorically proven or falsified; see Chapter 3). Models can put together the available information in ways that reflect a belief about how the system works and they, too, can produce hypotheses that can be followed up. However, in a set of five major ecological journals Grace (2019) reported that only 25% of articles used the word ‘hypothesis’, but that the proportion had increased from 15% over a 30-year period (Grace was responding to a criticism made of ecology by Peters (1991)). Betts et al. (2021) concluded that the frequency of hypotheses in eco-evolutionary publications over the same period was low and static. Based on this scant data, the testing of hypotheses does not seem to feature highly in ecological research, at least not explicitly stated.
The problem is that we tend to leave our search for explanations merely started: we collect descriptive data, we analyse them and we make an inference. Period. We are content to stop where we have an idea that is plausible, based on the limited available data; it may be correct but has not been rigorously investigated with all the methods available.
Alternative explanations for observations may not have been considered, or not yet, and papers describing these incomplete searches are published in many journals: not the best journals, perhaps, but the sheer number bias Ecology’s literature. Compare this with the standard of evidence in a legal system. If we gave expert evidence in a court case and we could only say that our ‘data suggest’ or that ‘there is a correlation’, does this fulfil the criterion of ‘beyond reasonable doubt’? No… and any student of logic would point out the weakness of such statements; as scientists, we need to raise our standards for the strength of evidence in ecological research.
10.3.2 Black box ecology
Much of ecology takes what we might call a ‘black box’ approach to science. It is indirect. We focus on the outputs of a system and then try to work backwards, attempting to postulate the processes inside the system which might explain our observations, but those processes are essentially unknown to us (Figure 10.1). We can’t see inside the box! We can change the inputs to the system and observe the way that the outputs change; this also might help us to understand how the system works. The alternative to a black box approach is a mechanistic approach, in which we observe the processes directly and try to build a picture (perhaps also a model) of the system itself: the ‘nuts and bolts’ of the system’s internal structure and how it is expected to function. Peters (1991) criticises this treating of ecological systems as if they are human-engineered machines, which they are not. They are more complex. Thus, our attempts to envisage the workings of ecological systems are doomed to be incomplete or naïve; again, a lack of stationarity in the processes we cannot see may threaten any understanding we have developed.
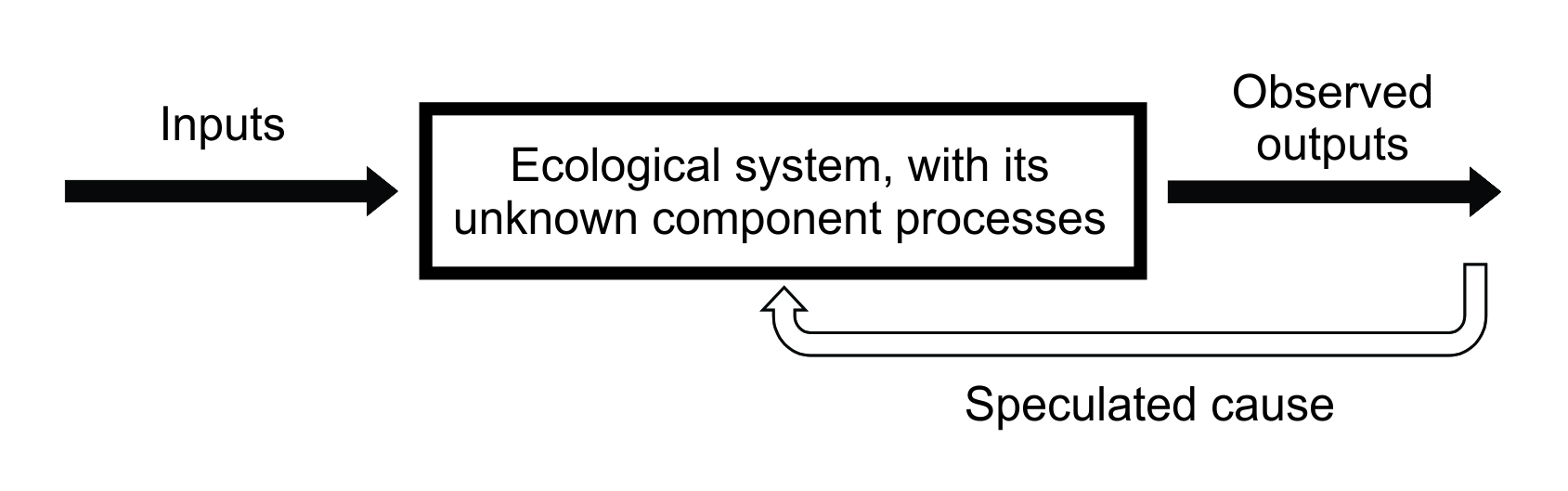
Figure 10.1 The black box approach to ecological understanding.
The problem with a black box approach is that we never know how far from reality our explanations are because we cannot examine the processes in the system. Our speculations provide explanations for the outcomes that may be sufficient but are not necessary. Other explanations are possible. Consider the example of the evolution of increased competitive ability (EICA) in invasive species. With this hypothesis, Blossey and Notzold (1995) suggested that the removal of a selection pressure on the invader that is present in the region of origin, such as specialist herbivores, should allow the evolution of traits to deal with new selection pressures in a new region. The removal of one selection pressure frees resources to address others. Specifically, ‘In the absence of herbivores, selection will favour genotypes with improved competitive abilities and reduced resource allocation to herbivore defence.’ Several researchers then looked for examples in which invasive plants were larger (size considered a surrogate for competitive ability) in introduced regions than in their putative source regions. The examples that were found were taken as evidence for the plausibility of the EICA hypothesis.
There are, however, other reasons for plants to grow bigger in their new locations, as Colautti et al. (2009) showed, such as adaptation to different growing season length. Even random processes, founder effects and genetic drift could produce some introductions that are larger than in the native region (and some that are smaller). Meta-analyses of similar data sets for native and introduced locations, whether they are biomass or competitive ability (by valid measurement, see Zhang et al. 2018), may show that individuals in introduced populations are often larger and from that point of view could support EICA, but they do not eliminate other possible mechanisms.
Meta-analyses may also be subject to a kind of sampling bias. It may have been easier to publish apparent support for EICA than studies that are non-significant or seem to contradict the hypothesis. For each suspected EICA case, we need to see evidence for the other conditions stipulated in the hypothesis: that the selection for herbivore defence is reduced, and defensive structures or secondary compounds are reduced in the introduced populations. These conditions must be all tested at the same time and in the same systems. We are trying to infer adaptation from a long-past event, and we require the assumption that the current post-adaptation introduced genotype’s mean phenotypes were originally identical to the current native-range genotypes that are being compared. This assumption might be false. Here is another case where non-stationarity in the form of unseen changes over time can undo our conclusions.
Black box approaches have also dominated ecological research on competition. Competition is defined as the differential access to resources in limited supply. Ecologists rarely investigate the processes that occur between individuals at a physiological level (i.e. their use of the resources in different aspects of development). Typically, we vary the intensity of competition by either altering the density and frequencies of individuals of coexisting species (resource demand) or the availability of particular resources in their shared supplies (resource supply). We then measure reproductive output and the rate of population growth. In mixtures of plant species, it was common to measure biomass and to calculate indices based on relative performances in mixture and in monoculture as surrogate measures of competition. Jolliffe (2001) listed more than 25 such indices. There was an intense argument about which indices to use and how such experiments should be conducted (see also Box 1.2), and attempts to interpret indices in quasi-scientific terms such as ‘aggressivity’.
There are some examples of competition research where the physiological processes themselves were studied, measured and modelled, particularly in the eco-physiological modelling of competing weed-crop systems (Kropff and van Laar 1993; Louarn and Song 2020), but these never became popular beyond agricultural systems. The tradition of focusing on outputs and trying to infer processes continues in studies of species introductions into complex assemblages of plants and functional types.
Seed dispersal is one area in which there has been a swing from black box ecology to more mechanistic approaches. Models of dispersal derived from basic fluid mechanics have been developed over several decades, culminating in the work of Nathan and Katul (2005). These studies focus on movement of individuals from point sources under specified conditions (projections as defined in Chapters 7 and 8, not predictions). A realisation from this work is that to predict dispersal distances requires an understanding of ‘movement ecology’ (Nathan et al. 2008), such as the rules governing the locomotion of an animal and meteorological vectors affecting dispersal. This has much in common with another process-based modelling approach, agent-based modelling (McLane et al. 2011), and is highly relevant to wildlife conservation (Fraser et al. 2018).
This discussion of the limitations of black box ecology may sound like a plea to re-establish physiological ecology into mainstream ecology. Perhaps and perhaps not! The black box approach may well be holding the discipline back in many areas. In cases like EICA, we will only make progress by the direct study of the evolutionary and ecological processes, rather than system outputs such as phenology, moving from speculation to evidence-informed predictions. Many ecological systems, however, might be too complex to allow anything other than a black box approach as Peters (1991) argued and which we hinted in Chapter 2 in discussing complex multilayer networks. Many concepts that have dominated community ecology relate to attempts to predict system output or overall behaviour from its structure. In community ecology (see Chapter 9) progress has been made by moving the focus from the description of community structure towards understanding processes. Peters (1991) suggested that we might ‘operationalise’ ecological concepts by placing them within testable theories, thus connecting them to hypotheses about the underlying processes. Ecosystems have long been studied at a process level, using simplifications that permit a focus on the physical-chemical processes rather than communities’ complexities.
10.3.3 Collection of supporting evidence
Ecologists have been engaged in a long struggle to find rules that are sufficiently general to apply throughout ecology (see discussions in Chapters 3, 5 and 9): whether or not these rules are laws of nature (we’ll postpone that discussion). If our ideas, derived from many studies in different systems and locations, are truly sound, then they should apply across the variation found in the natural world. General rules would allow us to predict function in systems not yet studied. In recent decades, meta-analyses (with their own, formal methodology) have tried to establish whether or not there is evidence for such generalisations. Studies announce (or deny!) generalisations in their titles: ‘Climate niche shifts are common in invasive species’ (Atwater at al. 2017); ‘Climate niche shifts are rare among terrestrial plant invaders’ (Petitpierre et al. 2012). Generalisations have even been boldly announced on the results of a single study, encouraged by the modern trend towards ‘banner headlines’ for article titles.
It is no surprise that some ecologists hunt the literature for studies that support their favoured idea, based on the thought that if most studies support the generalisation, it has greater credence, even if the support is not universal. The term context dependency refers to the fact that different systems or different regions can give different results (Chapter 5), but this overall vote-counting procedure risks misleading the ecology community. Logical problems emerge if it seems that not all studies have equal weighting.
A well-known source of error is ‘confirmation bias’, where studies in favour of the expectation are collected, but contrary studies are ignored or discredited. The proportion of studies that support the expectation will be overestimated. This bias may be inadvertent: even if a meta-analysis does its best to access all published studies, there may well be an under-representation of contrary studies in the literature. It has always been easier to get a positive (statistically significant) result published in a journal (‘publication bias’), rather than an unclear (non-significant) or contrary (significant) result. Confirmation bias can also enter into individual studies when researchers expect to see a particular outcome and their observations become biased. Examples have been suspected and reported particularly in animal behaviour research (Marsh and Hanlon 2007).
Something similar to confirmation bias occurs when the attention of the observer is caught by exceptions and these become overemphasised. The statement that ‘hybridisation is an important factor influencing adaptation in invasive species’, for example, is based on an observation that several significant invasions have involved hybridisation. If ‘important’ is treated as synonymous with ‘often’, depending on the definitions of ‘often’ or ‘common’, it may overstate the situation.
10.3.4 How can we improve what we do?
As in other walks of life, some of us are more adept at difficult things than others, and so there are a range of standards of ecological research. A systematic appraisal of our research outputs, if that were possible, would be unlikely to reveal the greatest frequency in the ‘most outstanding’ category. There would probably be a broad spread, perhaps with a significant proportion being far from the best possible (as has been shown in surveys of our applications of particular techniques). Even the best products of the most lauded ecologists will not be perfect; like the rest of us, they are trying to find their way in an inexact, complicated discipline. Some will choose one approach, some another; and each approach will have limitations and may produce different interpretations of the results. While the best studies are praised, many lesser studies also provide worthwhile information and are published, and our emerging scientists may be tempted to see the norm, rather than the very best, as the benchmark.
We can warn our colleagues of potential problems in ecological methods. Those practices that are regarded as strong or weak can be highlighted through education and communication, but for these efforts to be effective, receptivity is crucial. There must be a general appreciation by ecologists that suboptimal research is common and that we – not someone else – need to take action. Will the community of proud practitioners, whose research is published and widely read, accept this admission of weakness and embrace the need for concerted action? Both denial and ignorance are likely to stifle uptake of even the best advice.
Even when evidence of poor standards has been widely communicated (such as the poor standards of species distribution modelling or of statistical analysis and interpretation), remedial action has not followed. The publication of awareness papers in journals that highlight and explain the problems, and even the adoption of best practice guides by journals, seems to be ineffective in inspiring action by editorial boards (see Chapter 7).
The inability of the peer review system to filter out poor research practices and to discriminate between good and bad use of research techniques has been widely and frequently described.
Despite the huge investment of time in the peer review process, its statistical power is poor because of high variances among reviewers and small sample sizes (Cousens 2019). It is difficult to see how to improve the peer review system without greatly increasing our investment in it. It may always be an ineffective sieve for weak research because those assessing the work have the same level of expertise as those whose work is being judged. In the case of journal articles, the research has been completed, and so there is no opportunity for formative evaluation and iterative improvement. What will be published in the future reflects the standards of what is already published and not what the standards ought to be.
Rather than try to solve the problem too late in the process, can we be proactive? Perhaps we need a new approach to how the discipline of Ecology is taught. In the limited curriculum time available, we tend to focus on the state of ecological knowledge, but founded on textbooks that had their first drafts decades ago, often with methods and case studies that have historical rather than current relevance. It is important for students to have an appreciation for the historical development of ecology, so that they can learn from how our predecessors made progress and so that they can appreciate how that history constrains current thinking. Practical classes give students limited experience with a few classical and experiments and procedures. It may be that we need a new curriculum that focuses on how to conduct effective ecological research.
Many scientists have argued that we learn best from our mistakes, not just from our successes. We need to open students’ eyes early, to appreciate how easy it is to do ecology poorly. It would be useful to have resource material that illustrates poor practices and how methods can be misused and results misinterpreted, and examples of critiques of published research. Resource material could also explore what we might do, and the various choices and compromises we have to make when the real world departs from the ideal.
10.4 And, finally, so what?
If you were expecting this book to tell you how the entire discipline of Ecology can be made more effective, we will have disappointed you.
No matter how passionately some people call for new approaches to sweep through our discipline, that will not happen readily or swiftly. If it happens at all, it will take an extremely long time. The entrenched nature of our approaches and the complexity of Ecology’s disciplinary network ensure lasting inertia. Our journals will continue to publish descriptive studies that barely touch the surface of a problem, with weak inferences and inconsistent quality control. On the other hand, Ecology will continue to be populated also by outstanding projects and syntheses of great depth and novel insight.
Rather than trying to set the entire ecological research world to rights, it is perhaps more a matter of altering the balance. We can do this by anticipating, recognising and addressing the discipline’s weaknesses and broadening the appreciation of its strengths: by strengthening critique, discourse, communication and education. This still requires a groundswell of opinion that we need to do it. How will that be achieved?
Those who are interested in more altruistic actions, who are in privileged positions and with influence, need to pursue ways to make better education and training available, and to ensure that more of the appropriate opportunities are in place for all ecologists to synthesise, to challenge and to debate.
Collecting data is easy. Making speculations based on that data is easy. It will always be hard, however, to make significant intellectual progress in ecology, and it will always be harder for some of us than for others and in some fields of research than in others. It is the improvements in the individual actions of its practitioners, acting within and enabled by their multilayered network, that will result in more effective ecology. We need more explicit goals, the right questions, the most effective actions, the right data, the right analyses and the right interpretations. This requires careful deliberation, common sense and something that we might consider art.
Significant change to standards in ecology requires appropriate attitudes:
- acceptance that we are not experts in everything, even in simple things that we do routinely (there is an ever-present danger that we will make mistakes)
- perception that we need help (and cultivate effective collaborations) and that we need to educate ourselves from the literature that indicates the best use and the misuse of our techniques
- recognition of the powers of critique applied to everything that we do and everything that we read and hear (Gide 1952: ‘Believe those who are seeking the truth. Doubt those who find it.’)
- deliberately search for weaknesses in what we do or have done, rather than hoping that they will not be found
- preparedness to go against the trend of what our respected colleagues are doing
- realisation that we make assumptions (and use surrogates or other indirect approaches) in almost everything and that those assumptions need to be checked and shown to be appropriate
- recognition that black box approaches lead to weak inference and that we need to focus on biological, ecological or evolutionary processes and system function.
Perhaps above all, we need to grasp the challenge to raise our expectations, from the search for the merely plausible to the demonstration of the most probable (‘beyond reasonable doubt’).
Plausible explanations may often turn out to be correct, but the most effective, highest quality science does not stop at initial investigations and first-pass conclusions. It applies the most appropriate subsequent methods to assemble the most comprehensive evidence that we can.
In following this path, we may cover less of nature’s diversity, but the strength of our arguments and our progress on those things that we do study will be so much more powerful. We are more likely, in this way, to push the boundaries of our knowledge more effectively.
10.5 References
Anderson, S. C., P. R. Elsen, B. B. Hughes, et al. 2021. Trends in ecology and conservation over eight decades. Frontiers in Ecology and the Environment 19:274-282. doi:10.1002/fee.2320
Atwater, D. Z., C. Ervine, and J. N. Barney. 2018. Climatic niche shifts are common in introduced plants. Nature Ecology and Evolution 2:34–43. doi: 10.1038/s41559-017-0396-z
Baron, J. S., A. Specht, E. Garnier, et al. 2017. Synthesis centers as critical research infrastructure. BioScience 67:750–759. doi:10.1093/biosci/bix053
Betts, M. G., A. S. Hadley, D. W. Frey, et al. 2021. When are hypotheses useful in ecology and evolution? Ecology and Evolution 11:5762-5776. doi:10.1002/ece3.7365
Blossey, B., and R. Nötzold. 1995. Evolution of increased competitive ability in invasive nonindigenous plants: a hypothesis. Journal of Ecology 83:887-889.
British Ecological Society. 2022. Future of ecological research in the UK. https://www.britishecologicalsociety.org/policy/future-of-ecological-research-in-the-uk/ accessed 27 October 2022.
Carmel, Y., R. Kent, A. Bar-Massada, et al. 2013. Trends in ecological research during the last three decades – A systematic review. PLoS ONE 8:e59813. doi:10.1371/journal.pone.0059813
Colautti, R. I., J. L. Maron, and S. C. H. Barrett. 2009. Common garden comparisons of native and introduced plant populations: latitudinal clines can obscure evolutionary inferences. Evolutionary Applications 2:187-199. doi:10.1111/j.1752-4571.2008.00053.x
Cousens, R. 2017. Do we argue enough in ecology? Bulletin of the British Ecological Society 48:58-61.
Cousens, R. 2019. Why can’t we make research grant allocation systems more consistent? A personal opinion. Ecology and Evolution 9:1536–1544. doi:10.1002/ece3.4855
Currie, D. J. 2019. Where Newton might have taken ecology. Global Ecology and Biogeography 28:18-27. doi:10.1111/geb.12842
Dale, M. R. T, and M. -J. Fortin. 2014. Spatial analysis: A guide for ecologists, 2nd edition. Cambridge: Cambridge University Press. doi:10.1017/CBO9780511978913
Felker‐Quinn, E., J. A. Schweitzer, and J. K. Bailey. 2013. Meta‐analysis reveals evolution in invasive plant species but little support for Evolution of Increased Competitive Ability (EICA). Ecology and Evolution 3:739-751. doi:10.1002/ece3.488
Fraser, K. C., K. T. A. Davies, C. M. Davy, et al. 2018. Tracking the conservation promise of movement ecology. Frontiers in Ecology and Evolution 6:150. doi:10.3389/fevo.2018.00150
Gide, A. 1952. Ainsi-soit-il, ou: Les jeux sont faits. Paris: Gallimard. Grace, J. 2019. Has ecology grown up? Plant Ecology and Diversity 12:387-405. doi:10.1080/17550874.2019.1638464
Guo, Q., S. Jin, M. Li, et al. 2020. Application of deep learning in ecological resource research: Theories, methods, and challenges. Science China Earth Sciences 63:1457–1474. doi:10.1007/s11430-019-9584-9
Halpern, B. S., E. Berlow, R. Williams, et al. 2020. Ecological synthesis and its role in advancing knowledge. BioScience 70:1005-1014. doi:10.1093/biosci/biaa105
Jolliffe, P. A. 2001. The replacement series. Journal of Ecology 88:371-385. doi:10.1046/j.1365-2745.2000.00470.x
Kropff, M. J., and H. W. van Laar. 1993. Modelling crop-weed interactions. Wallingford: CAB International.
Louarn, G., and Y. Song 2020. Two decades of functional–structural plant modelling: now addressing fundamental questions in systems biology and predictive ecology. Annals of Botany 126:501-509. doi:10.1093/aob/mcaa143
Marsh, D. M., and T. J. Hanlon. 2007. Seeing what we want to see: Confirmation bias in animal behaviour research. Ethology 113:1089–1098. doi:10.1111/j.1439-0310.2007.01406.x
McCallen, E., J. Knott, G. Nunez-Mir, et al. 2019. Trends in ecology: Shifts in ecological research themes over the past four decades. Frontiers in Ecology and the Environment 17:109–116. doi:10.1002/fee.1993
McLane, A. J., C. Semeniuk, G. J. McDermid, et al. 2011. The role of agent-based models in wildlife ecology and management. Ecological Modelling 222:1544-1556. doi:10.1016/j.ecolmodel.2011.01.020
Nathan, R., and G. G. Katul. 2005. Foliage shedding in deciduous forests lifts up long-distance seed dispersal by wind. Proceedings of the National Academy of Science of the United States of America 102:8251-8256. doi:10.1073/pnas.050304810
Nathan, R., W. M. Getz, E. Revilla, et al. 2008. A movement ecology paradigm for unifying organismal movement research. Proceedings of the National Academy of Science of the United States of America 105:19052-19059. doi:10.1073/pnas.0800375105
Peters, R. F. 1991. A critique for ecology. Cambridge: Cambridge University Press
Petitpierre, B., C. Kueffer, O. Broennimann, et al. 2012. Climatic niche shifts are rare among terrestrial plant invaders. Science 335:1344-1348. doi:10.1126/science.121593
Sutherland, W. J., R. P. Freckleton, H. C. J. Godfray, et al. 2013. Identification of 100 fundamental ecological questions. Journal of Ecology 101:58-67. doi:10.1111/1365-2745.12025
UK Research and Innovation. 2021. Sandpits. https://beta.ukri.org/councils/epsrc/guidance-for-applicants/types-of-funding-we-offer/transformative-research/sandpits/ accessed 19 September 2022.
Zhang, Z., F. Zhou, X. Pan X. et al. 2018. Evolution of increased intraspecific competitive ability following introduction: The importance of relatedness among genotypes. Journal of Ecology 107:387-395. doi:10.1111/1365-2745.13016