Topic 9 Cell Junctions and Cell Adhesions
Figure 9.1: Epithelial and Connective Tissue Connecting Cells
The above figure displays two ways in which cells can be bound in an organism.
9.1 Cell Junctions
Figure 9.2: Four Main Types of Junctions
Cell junctions are a form of physical contact with other cells - there are four main types of junctions:
- Anchoring junctions
- Occluding junctions
- Channel-forming junctions
- Signal-relaying junctions
Figure 9.3: Classifications of the Four Main Types of Junctions
Also note the above table for multiple types of junctions.
Figure 9.4: Junctions in an Animal Cell
In theory, these junctions are all present in an animal cell like shown in the above figure.
9.2 Anchoring junctions
Such junctions have four main components:
- Adhesion protein
- Extracellular ligand
- Cytoskeleton
- Anchor proteins
Two kinds of anchoring junctions are examined in BS2004:
Adherens Junctions
- Adhesion protein = classical cadherins
- Extracellular ligand = cadherins in neighboring cells
- Cytoskeleton = actin filaments
- Anchor proteins = \(\alpha\)-catenin, \(\beta\)-catenin p120-catenin, and so on.
Desmosome junctions
- Adhsion protein = desmoglein and desmocolin
- Extracellular ligand = desmoglein and desmocolin in neighboring cells
- Cytoskeleton = intermediate filaments
- Anchor proteins = plakoglobin, plakophilin, desmoplakin
9.2.1 Classical cadherins
Figure 9.5: Structure of a Cadherin
These include E-cadherins, N-cadherins, P-cadherins, and VE-cadherins.
Figure 9.6: Cadherin Adhesion Using Calcium Ions
All cadherins need Ca2+ ions for binding and are localized to cell-cell junctions.
9.2.1.1 Selective assortment of cells
When cells express E-cadherin and N-cadherin are mixed, they sort out and aggregate separately. Hence, this suggests that cadherins prefer binding to their own types.
Figure 9.7: Cadherin in Cell Sorting
This property of cadherins can be used to explain the assembly of cells to form tissues.
9.2.1.1.1 Formation of the nervous system
Figure 9.8: Formation of the Nervous System in Terms of Cadherins
The above figure shows how cadherins are involved in the formation of the nervous system.
Figure 9.9: Formation of the Nervous System in Terms of Cadherins
Some cells that are initially a part of the embryo alter their adhesive properties to a form a neural crest on the upper surface of the neural tube.
The cells then migrate to form a variety of cell types throughout the embryo. In the above graphic, ganglia: clusters of nerve cells in the peripheral nervous system, are formed. Some neural crest cells also differentiate into neurons and satellite cells.
9.2.1.2 Maintenance of spindle orientations
Figure 9.10: Linkage of Cadherin to the Actin Cytoskeleton
Cadherin is linked to an actin cytoskeleton via catenins.
While it is not fully understood why cadherin-actin interactions are as such, there are one of several hypotheses:
Cadherin’s cytoplasmic domain binds both p120 catenin and \(\beta\)-catenin which then associates with \(\alpha\)-catenin: a protein mutually exclusive with F-actin interactions. However, its dimer reacts with F-actin.
\(\alpha\)-catenin interacts with vinculin - a protein that can bind to F-actin.
Figure 9.11: Vinculin Linking
Myosin VI can interact with E-cadherin cytoplasmic tails, linking actin filaments to cadherin receptors.
9.2.1.3 Roles of cadherins and the actin cytoskeleton
At the cellular level, Actin assembly at the adherens junctions make cell-cell junctions strong. The disassociation of such cell-cell adhesions are also important in embryo growth.
Cadherins and the actin cytoskeleton also play roles in myogenesis and the development of the nervous system.
9.2.2 Epithelial mesenchymal transition (i.e., EMT)
EMT is the transdifferentiation / transition of epithelial cells to mesenchymal cells.
Note the comparisons between epithelial cells and mesenchymal cells:
cell | Trait1 | Trait2 | Trait3 | caption |
---|---|---|---|---|
Epithelial Cells | Cell-cell adhesion | Planar / apical-basal polarity | High e-cadherin expression | Comparison Between Epithelial and Mesenchymal Cells |
Mesenchymal Cells | Loss of cell-cell adhesion | loss of polarity | High n-cadherin expression | Comparison Between Epithelial and Mesenchymal Cells |
EMT generally happens in would healing and embryo development. Metastasis during cancer progression may also involve EMT.
9.2.3 Desmosomal cadherins
Figure 9.12: Desmosomal Cadherins are Linked to Intermediate Filaments
Figure 9.13: Desmosomal Cadherins are Linked via Proteins from the Catenin Family
Note the above graphics for this section.
9.3 Desmosomes
Figure 9.14: Model of a Desmosome
Observe the general structure of a desmosome in the above graphic. Recall that desmosomes are connected to intermediate filaments via proteins from the catenin family. The main function of a desmosome is to provide mechanical strength.
9.4 Occluding Junctions
Figure 9.15: Occluding Junctions in Theory and in Practice
These are also known as tight junctions - they form a seal between cells and allow the epithelium to serve as a barrier to solute diffusion.
Figure 9.16: ZO-1-Stained Murine Hepatocytes in Tight Junctions
In the above graphic, the protein ZO-1 (found in tight junctions) appears green and DAPI appears blue.
In tight junctions, glucose is transported via a symporter at the apical cell membrane before being transported via facilitated diffusion at the basolateral membrane.
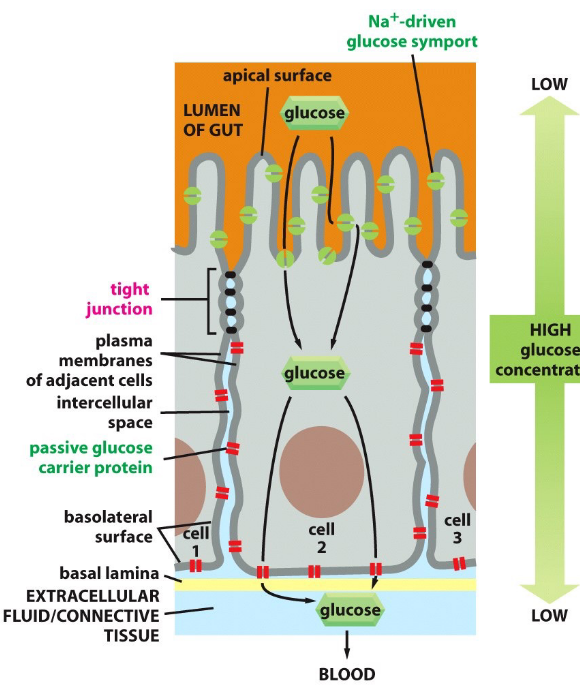
Figure 9.17: Glucose Transportation Across Cell Membranes
Tight junctions are thought to confine transport proteins to appropriate locations in cells to serve as diffusion barriers.
Tight junctions also stop glucose from flowing back into the epithelial side of the lumen.
In short, one can say that tight junctions perform the following two functions:
- Regulating paracellular permeability
- Forming the apical basolateral diffusion barrier
9.4.1 Proteins at tight junctions
Some proteins are:
Transmembane proteins
- MARVEL domain proteins: occludin, tricellulin MARVELD3
- Claudins
- BVES - Blood Vessel Epicardial Substance
- JAMs - Junctional Adhesion Molecules
- CRB3 - Crumbs Homologue 3
- Immunoglobulin-type adhesion proteins
Cytoplasmic plaque
- Adaptor proteins and linkers: ZO1, ZO2, ZO3, cingulin.
- Polarity proteins: PAR3, PAR6, etc.
- Signalling proteins: aPKC, CDC42, RAC, etc.
Claudin is the main functional component in sealing stands (which include claudin and occludin).
9.4.2 Diseases in tight junctions
Mutations in Claudin 16 and Claudin 19 can cause hypomagnesaemia (i.e., renal magnesium wasting) - an illness that results from a lack of magnesium resorption in kidney cells.
Tight junction proteins are also a target of pathogens - these interactions also result in the loss of an epithelial barrier from the disassociation of junctions.
The CagA protein of Helicobacter pylori associates with the ZO-1-JAM-A complex of the tight junction and impairs with epithelial functions.
9.5 Channel-Forming Junctions
In plant cells, these are known as plasmodesmata. Otherwise, they are known as gap junctions in animal cells.
Such junctions also allow cells to exchange small molecules, but not macromolecules. These junctions do not allow for electricity to pass through and are also very dynamic structures that readily assemble and disassemble.
9.5.1 Gap junctions
Figure 9.18: Structure of a Gap Junction
Gap junctions are formed via assemblies of proteins called connexons. Each connexon is made up of six subunits.
9.5.1.1 Determining the size of a gap junction
Figure 9.19: Dye Injection into a Cell
First, inject fluorescent molecules of various sizes into one of two cells that are linked by gap junctions.
Second, we then detect for the fluorescent markers in the other cell.
From this, we found that only molecules that are 1000 daltons or less in size can pass through gap junctions.
9.5.2 Signal-relaying junctions
Figure 9.20: A Textbook Depiction of a Synapse
A synpase is the space between two ends of a neuron.
Figure 9.21: Scaffold Proteins Holding Together a Synpase
Scaffold proteins are used to hold various components of the synapse together.
9.6 Extracellular Matrix
The basal lamina is found in epithelial tissues. The basal lamina is a thin sheet (40 to 120 nm thick) that underlies all epithelia in multi-cellular animal cells.
It also wraps around other types of cells (e.g., muscle cells)
Figure 9.22: Locations of the Extracellular Matrix
The extracellular matrix is also found in connective tissues (e.g., bone, blood, tendons, and the dermis).
9.6.1 Components of the basal lamina
Figure 9.23: Molecular Structure of the Basal Lamina
There are two main components:
Fibrous proteins
Glycoproteins (e.g., laminin, type IV collagen, nidogen)
Polysaccharide chains
These are linked to core proteins to form proteoglycans.
9.6.2 Extracellular matrix of animal cells
Figure 9.24: Extracellular Matrix of Animal Connective Tissues
The macromolcules here are similar to those found in the basal lamina:
Glycosaminoglycan (i.e., GAG)
These are usually covalently linked to proteins to form proteoglycans (e.g., chondritin sulfate, heparan sulfate).
Non-proteoglycan polysaccharides
For instance, hyaluronic acid.
Fibrous proteins
For instance, collagen and elastin
9.6.2.1 Cell types involved in the production of an extracellular matrix
Some cell types include:
- Fibroblasts in connective tissues
- Chondrocytes in cartilage
- Osteoblasts in bone
Cells help by organizing collagen fibers by exerting tension on the matrix.
9.6.3 Cell matrix adhesion
There are two main ways that this can happen:
Integrin adhesion complex
This links the extracellular matrix to the actin cytoskeleton
Hemidesmosome
This links the extracellular matrix to the intermediate filaments.
9.7 Integrin Family of Adhesion Receptors
These proteins have a heterodimeric cell surface of 24 \(\alpha\) and \(\beta\) subunits. There are at least 24 known integrin receptors from combinations of 18\(\alpha\) and 18\(\beta\) subunits.
Figure 9.25: Family of Integrin Receptors
Upon binding to the extracellular matrix, integrin receptors on the membrane form focal adhesions via recruiting other proteins through signalling and adaptor proteins.
9.7.1 Integrin activation
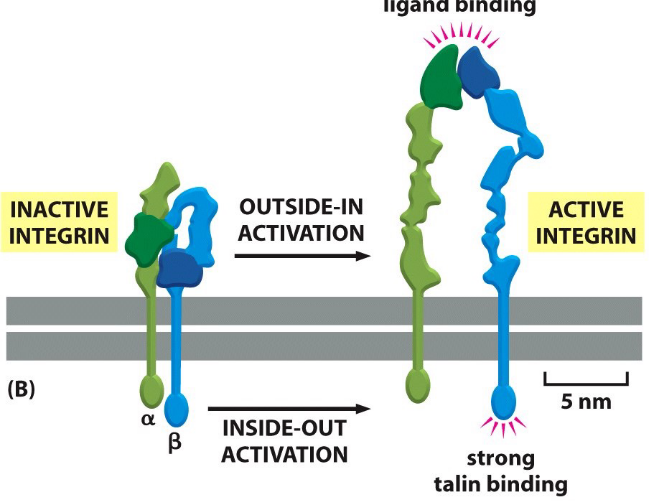
Figure 9.26: Conformations of Ligand Binding
Activated integrins can adopt extended conformations:
Outside-in conformation
This happens wheh integrins bind to the extracellular matrix
Inside-out activation
Figure 9.27: Inside-Out Activation of Integrins
This includes activation by other signalling pathways inside the cell.
Figure 9.28: Diseases Caused by Integrin Defects
Also note the diseases caused by defects in integrin.
9.8 Focal Adhesions
These are large protein complex via which the actin cytoskeleton of a cell is connected to the extracellular matrix. Focial adhesions are also formed at the site of integrin binding and clustering.
Figure 9.29: Formation of Focal Adhesion Complexes
More than 100 proteins have been found at focal adhesions - for instance, vinculin, paxillin, \(\alpha\)-actinin, and talin.
9.8.1 Models for forming nascent adhesions
First model
Such adhesions begin via the binding of integrins to extracellular matrix proteins which form new adhesion complexes on the clustered integrin cytoplasmic domains. These complexes are made up of talin, vinculin, \(\alpha\)-actinin, and actin.
Second model
These adhesions are formed via actin polymerization; viculin binds to the actin-related protein 2 / 3 (i.e., APR 2/3) complexes than then bind integrins. Like the first model, adhesion maturation happens via increased tension and bundling and cross-bridging of actin filaments.
9.8.2 Integrin-mediated survival versus apoptotic pathways
Integrins can be responsible for pro-survival and pro-apoptotic signals. Which pathway is more active depends on the ligation status of the surface.
In pathways where most integrins are ligated, a pro-survival pathway is initiated via increased amounts of nuclear factor-\(\kappa\)B or PI3K-AKT activity, decreased p53 activation, and an increased expression of BCL-2 and FLIP.
Cooperative signalling between growth factor receptors and integrins also differentially activates Raf, leading to distinct mechanisms of cell survival.
Signalling through integrin \(\alpha\)v$$3 and the fibroblast growth factor promotes the phosphorylation of Ser338 and Ser339 of Raf, hence protecting cells from the intrinsic pathway of apoptosis. Integrin \(\alpha\)v$$5 and vascular endothelial growth factor receptor 2 phosphorylate Tyr340 and Tyr341 of the Raf to prevent apoptosis via the extrinsic pathway.
9.8.3 Cell death as a consequence of a loss of adhesion
Unligated integrins begin the cleavage of caspase 8 in adherent cells, triggering apoptosis via integrin-mediated death (i.e., IMD).
When adhesion is completely lost, cell death occurs via anoikis. Apoptosis due to anoikis may happen intrinsically or extrinsically.