Chapter 8 Glycogen Synthesis
The human brain requires 120 grams of glucose per day out of the 160 grams of glucose that is needed by the body.
Fortunately, glycogen reserves can provide up to 190 grams of glucose and bodily fluids can provide up to 20 grams: the body already contains about one day’s worth of glucose stores!
However, when glucose is depleted (e.g., fasting or prolonged exercise), glucose must be synthesized from other sources; gluconeogenesis provides glucose to the body’s tissues by promoting the degradation of glycogen stores.
8.1 What is Glycogen?
This is the main form of storage for glucose in most prokaryotic cells (not including plants). It is a large and highly branched polysaccharide that has glucose units joined by α-1,4 and α-1,6 glycosidic bonds:
Figure 8.1: Structure of Glycogen
Nevertheless, glycogen is stored inside cytosol granules of the liver and the muscle; in mammals, it accounts for up to 10% of muscle mass and 2% of liver mass.
Glycogen degradation in the liver is needed to maintain blood glucose levels, whereas glycogen degradation in the muscles is needed to provide energy for movement.
8.2 Glycogen Metabolism Overview
Three key enzymes are needed for glycogen metabolism:
- Glycogen phosphorylase
- Glycogen synthase
- (De)Branching enzymes
Figure 8.2: Enzymes for Glycogen Metabolism
Glycogen degradation and synthesis happens in the cytosol; these reactions typically happen at the free, branching ends of glycogen (i.e., their nonreducing ends).
Figure 8.3: Non-Reducing Ends of Glycogen
The large amount of glycogen branch points gives rise to a highly efficient mechanism to release and store glucose.
8.3 Glycogenesis
Figure 8.4: Glycogenesis in a Nutshell
Note that although the above reaction is highly reversible, the hydrolysis of pyrophosphate pushes the reaction the right!
8.3.1 Glycogen synthase is a key enzyme in glycogenesis
Glycogen synthase can only add a glycosyl residue if and only if the polysaccharide chain already has four or more residues.
Figure 8.5: Mechanism of Glycogen Synthesis
The activity of this enzyme is modified by covalent modification and allosteric ligand alterations. Multi-site phosphorylation of this enzyme changes the net charge of this enzyme at the terminal N and C ends.
8.3.1.1 Phosphorylation of glycogen synthase
This enzyme is phosphorylated by three major enzymes:
- Glycogen synthase kinase 3 (GSK3)
- Protein kinase A (PKA)
- Casein kinase (CKII)
Insulin activates glycogen synthase b via inhibiting GSK3 activity through activating a phosphoprotein phosphatase (PP1 in muscle, another phosphatase in the liver):
Figure 8.6: Insulin Activating Glycogen Synthase B
It is also key to note that glucose-6-phosphate favors the dephosphorylation of glycogen synthase by binding to it, thereby promoting a conformation that favors the binding of PP1.
Glycogen synthase also requires a primer - glycogenin.
8.3.2 Glycogenin - a primer for glycogen synthase
Glycogenin is a 37 kDa big homodimer and catalyzes two distinct reactions:
- The initial attack of C1 on UDP-glucose by the OH group of Tyr194.
- The attack of another UDP-glucose’s C1 by the C4-OH of the terminal glucose
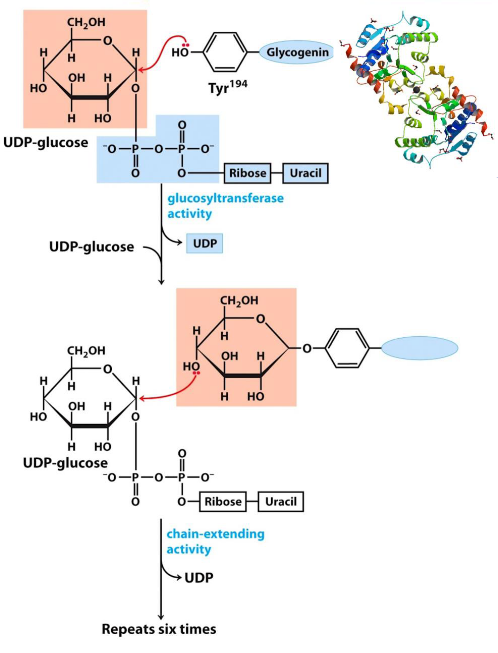
Figure 8.7: Glycogenin Catalyzes these Reactions
The above two reactions repeat themselves to form a glycogen molecule with eight glucose molecules that are attached by α-1,4 glycosidic linkages.
Figure 8.8: Structure and Function of Glycogenin
8.3.3 Hormonal regulation of fuel metabolism
The hormones insulin, glucagon, and epinephrine (from the adrenal glands in the kidneys) is used to maintain blood glucose levels in humans.
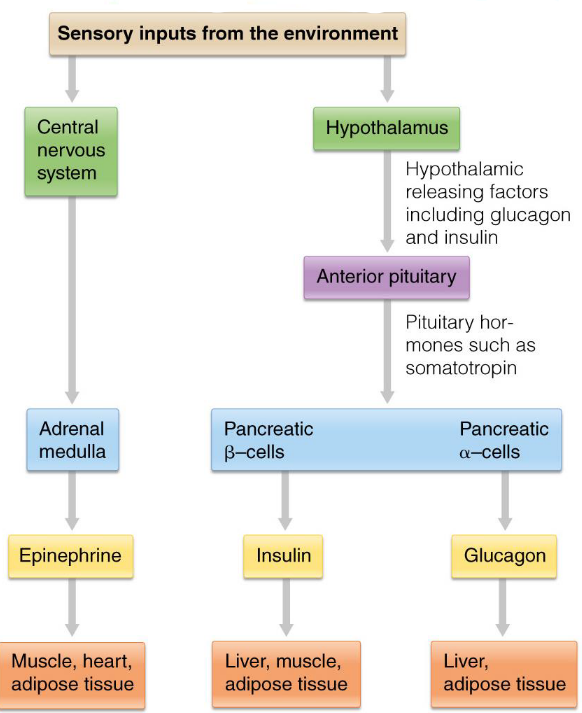
Figure 8.9: Hierarchical Control of Hormones
The (anterior) pituitary gland is the first target for most hormones; pituitary hormones then act on secondary targets.
Neural stimulation of the adrenal medulla releases epinephrine.
8.3.4 Glucose activates glycogen synthase
As mentioned previously, glucose also promotes the dephosphorylation of glycogen synthase; it does this via binding to glycogen phosphorylase a.
Figure 8.10: Glucose Binding to Glycogen Synthase
This induces a conformational change that favors the dephosphorylation to glycogen phosphorylase b, hence allowing for PP1 to bind to the enzyme (shown above).
8.4 Glycogenolysis
This reaction degrades glycogen in three steps:
- Releasing glucose-1-phosphate from glycogen
- Rearranging remaining glycogen to permit continued breakdown
- Converting glucose-1-phosphate into glucose-6-phosphate for further metabolism
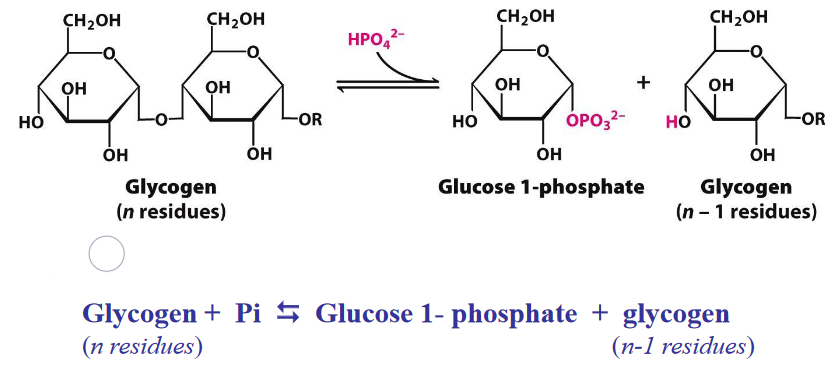
Figure 8.11: Glycogen Breakdown
The equilibrium of the above reaction is shifted towards the right because of the high amount of Pi; phosphorolytic cleavage is advantageous because sugars are phosphorylated.
8.4.1 Additional enzymes required for glycogenolysis
Figure 8.12: Additional Enzymes Needed for Glycogenolysis
Transferase transfers three glycogen molecules from one outer branch to another. α-1,6-glucosidase is needed for the hydrolytic activity of α(1,6)-linked glucose.
8.4.1.1 α-1,6-glucosidase and phosphoglucomutase
Figure 8.13: alpha-1,6-glucosidase
This is a single, 160 kD polypeptide chain.
Phosphoglucomutase converts glucose-1-phosphate into glucose-6-phosphate (as you may recall from chapter 3 of this website).
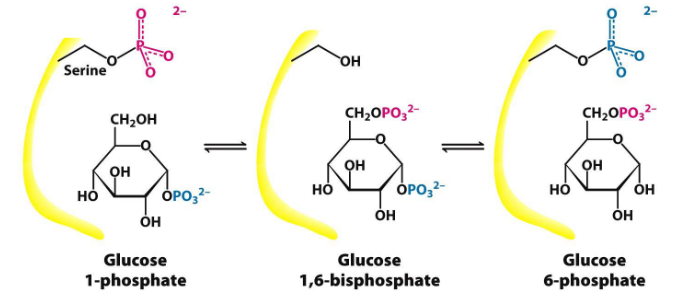
Figure 8.14: Phosphoglucomutase Mechanism
First, a phosphoryl group is transferred from the enzyme to the substrate; a different phosphoryl group is then transferred back to the enzyme to restore the enzyme to its initial state.
8.4.1.2 Glucose-6-phosphatase
This is an enzyme present in the liver - it cleaves phosphoryl groups to form free glucose and orthophosphate.
Glucose-6-phosphatase is absent from most tissues.
Glucose-6-phosphate is dephosphorylated prior to being moved out from the liver; this is because glucose-6-phosphate is a polar molecule (i.e., negatively charged) and cannot pass through the cell membrane via simple diffusion:
Figure 8.15: Glucose-6-Phosphate Mechanism
8.5 More About Glycogen Phosphorylase
Glycogen phosphorylase needs pyridoxal phosphate (i.e., PLP) as a cofactor.
The aldehyde group of this coenzyme forms a Schiff base with a specific lysine side chain of the enzyme:
Figure 8.16: PLP as a Cofactor
The 5’ phosphate group of PLP serves as a proton donor and then as an acceptor.
Furthermore, a bound HPO42- group (in red) favors the cleavage of the glycosidic bond by donating a proton to the departing glucose (in black).Figure 8.17: More About Phosphorylase
Furthermore, glycogen phosphorylase is also regulated by several allosteric enzymes that signal the energy state of the cell by reversible phosphorylation (this state of the cell is responsive to hormones such as insulin, glucagon, and epinephrine).
8.5.0.1 Phosphorylase a and b
The position of equilibrium between the T and R form of glycogen phosphorylase is responsive to conditions inside the cell.
The R state is favored by glycogen phosphorylase a; the T state is favored by glycogen phosphorylase b.
Figure 8.18: T and R States of Glycogen Phosphorylase
The transition from the T to the R state is associated with structural changes in α helices that move out of the active site of each subunit.
Phosphorylase kinase catalyzes covalent modification of glycogen phosphorylase.
8.5.0.2 Regulation by AMP, ATP, and G6P
Figure 8.19: States of Muscle Phosphorylase B
Muscle phosphorylase b is only active in the presence of high concentrations of AMP (which binds to a nucleotide-binding site and stabilizes the R state of phosphorylase b)
ATP is a negative allosteric effector and combines with AMP to favor the T state.
G6P also inhibits muscle phosphorylase b.
8.5.1 Muscle glycogen phosphorylase
This has two forms: an a form and a b form.
The a form is the active form and has two subunits, each of which has two phosphorylated serine residues.
The b form is the inactive form and is present in all resting muscle tissues. It is structurally identical to the a form, albeit the serine residues are not phosphorylated. When ATP and glucose-6-phosphate levels are high, the b form.
The conversion between the a and the b form is catalyzed by protein phosphatase I (and epinephrine).
8.5.2 Liver glycogen phosphorylase
The primary structure of glycogen phosphorylase in the liver and in muscles are 90% similar.
Liver phosphorylase a (not b) is the most responsive to the T-R state transition.
When glucose binds, the R state of the enzyme is shifted to the T state, hence deactivating the enzyme.
Figure 8.20: Liver Glycogen Phosphorylase
8.6 Phosphorylase Kinase Activation
The fully active form of the enzyme has four subunits: α, β, γ, δ - the γ subunit is the source of catalytic activity; all other subunits are regulatory subunits.
The active form of this enzyme has a mass of 1200 kD; phosphorylase kinase also has an inactive form!
Figure 8.21: Regulation of Phosphorylase Kinase
The β subunit is phosphorylated by cAMP and dependent PKA. The δ subunit, on the contrary, is calmodulin: a calcium sensor that stimulates many enzymes.
Phosphorylase kinase has the highest activity only after activation of the β and the γ subunits.
8.6.1 Cascade mechanism of epinephrine and glucagon
Figure 8.22: Cascade Reaction
When epinephrine acts on a myocyte or glucagon acts on a hepatocyte, there is a rise in cAMP concentrations (thereby activating PKA).
PKA then activates phosphorylase b kinase which then activates glycogen phosphorylase.
This cascade reaction can then provide glucose for muscle contraction and for maintaining blood glucose levels.