Chapter 8 Controlling Microbes
8.1 Definitions of Commonly-Used Terminology
Antiseptics are compounds that are applied on body surfaces to either destroy or inhibit pathogens (from growing).
Sanitation is the act of reducing microbe levels to a “safe” level.
Disinfection refers to the destruction or removal of pathogens, but not bacterial endospores. Disinfection typically occurs on inanimate objects.
Sterilization is the complete removal or destruction of microorganisms; this technique is also used on inanimate objects.
Chemotherapy is the usage of chemicals to either kill or inhibit microbes from growing within host cell tissues.
Figure 8.1: Effectiveness (in log(microbe population) of Various Sterilization Techniques
8.2 Microbial Death and Antimicrobial Agent Activities
Micro-organisms are not instantly killed; rather, their death (at a population level) occurs exponentially, and the efficacy of their deaths is measured via the decimal reduction time: the time needed for a 1 log kill (i.e., to kill 90% of the population).
Persister cells are the toughest microbes to kill as they are viable, but non-culturable (i.e., “VBNC”), have a low metabolic rate, and don’t divide (and hence, are unable to form a colony forming unit). Furthermore, VNBC cells have the ability to reproduce and cause infection under specific conditions
8.2.1 What are some conditions that influence an antimicrobial agent’s activity?
A larger population size would need more time for disinfection / sterilization (for obvious reasons).
Also, depending on the population composition, different agents may not work that well on certain species.
The concentration of the antimicrobial also influences how well the agent kills microbes.
Then, the duration of exposure also influences how successful an antimicrobial agent is in killing microbes.
A higher temperature kills microbes as well (e.g., pasteurization).
Lastly, the local environment also impacts the effctiveness of an antimicrobial agent: this includes the pH, viscosity, and the organic matter composition of the environment. As a general rule of thumb, organisms in biofilms are more resistant to many antimicrobial agents.
8.3 Physical Control of Microbes
8.3.1 Moist Heat
This is a very effective way of killing microbes, including but not limited to viruses, fungi, bacteria, and protists
Figure 8.2: Moist Heat Killing Microbes
Moist heat degrades nucleic acids, denatures proteins, and disrupts membranes via hydrolysis. As mentioned many chapters ago, spores are more resistant to heat and moisture than vegetative cells (e.g., Bacillus spores can resist up to 111 degrees Celsius, but live B. subtilis B692 cells can only take up to 57 degrees Celsius)!
8.3.2 Autoclaving
Figure 8.3: Autoclaving Kills Microbes
An autoclave uses steam sterilization to kill microbes. It is typically performed at temperatures of 100 degrees Celsius and above under high pressure; however, all instruments must be “opened” prior to being autoclaved (e.g., if one has a flask, the flask must be uncovered lest the autoclaving be ineffective)!
Autoclaving is an effective sterilization technique against all microorgranisms (spores included).
Quality control strips (i.e. strips with the thermophile Geobacillus stearothermophilus) are also used for quality control.
The magic formula for autoclaving is: 121 degrees Celsius at 103.5 kPa for 15 minutes (the time increases for larger volumes).
8.3.2.1 A general guideline for sterilization times based on volume of liquid solutions:
Volume.in.ml | Time.in.mins |
---|---|
10 | 15 |
95 | 15 |
1500 | 30 |
6750 | 70 |
However, one should realize that it is not always possible to follow this guide. Different mediums (when autoclaved) may caramelize!
At any rate though, it is important to have an optimally functioning autoclave to keep autoclaving times as minimal as possible.
8.3.2.2 Why is autoclaving so important?
Autoclaving is how microbiologists ensure that their tools are sterile. Otherwise, microorganisms cannot be maintained.
Furthermore, aseptic techniques and sterilization is one of the first things that you’ll be ask about when applying for a job in the industry.
8.3.3 Pasteurization
This was a process invented by scientist Louis Pasteu in 1864. Not only did pasteurization allow food to be stored for a longer period of time, but it also prevented many diseases.
Pasteurization utilizes controlled heating to reduce the population of microbes, thereby slowing the food spoilage process via reducing the total amount of organisms present.
Pasteruziation is also applied to diary products (e.g., milk and cheese), beer, and other beverages.
8.3.3.1 How should we pasteurize our products?
This really depends on the product at hand; as mentioned in the chapter on food microbiology, pasteurization timings can affect the flavor of the end product!
However, there are several traditional pasteurization routines:
- High temperature, short time treatment (i.e., HTST): 72 degrees Celsius for 15 minutes.
- Low temperature, long time treatment (i.e., LTLT): 63 degrees Celsius for 30 minutes.
In the case of milk, HTST not only preserves the flavor of the milk, but also maintains the nutrient composition of the milk.
8.3.4 Dry heat sterilization
This is less effective than moist heat sterilization; furthermore, such a sterilization technique is not applicable to the food industry. Not only does it oxidize cellular constituents, but it also denatures proteins (e.g., unintentionally cooking eggs).
Dry heat sterilization occurs at 160 - 170 degrees Celsius for 2 to 3 hours and are useful for solids that are affected by moist heat, but not high temperature (e.g., semolina flour).
8.3.5 Ionizing radiation
As mentioned in the food microbiology as well, γ radiation penetrates deep into objects, hence destroying bacterial endospores in a non-radioactive manner.
γ radiation is generally used to sterilize and pasteurize antibiotics, hormones, sutures, plastic disposable supplies, and food (e.g., meat, eggs, etc).
8.3.6 UV radiation
Radiation with a wavelength of 260 nm is bacteriocidal; DNA absorbs radiation at 260 nm, consequently creating thymine dimers and preventing replication and transcription.
However, UV radiation does not penetrate glass, dirt films, water, and is only limited to surface decontamination.
Interestingly, UV radiation has been used for water treatment.
8.4 Chemical Antimicrobial Agents
In 1867, Joseph Lister suggested using carbolic acid (or phenols) to disinfect surgical patients and wounds; it is worth noting that the disinfectant must be effective against a wide variety of microbes and around the presence of organic matter.
However, the overuse of antiseptics such as triclosan has lead to triclosan and possibly antibiotic-resistant bacteria.
8.4.1 Phenolics
These are compounds that are generally used as laboratory and hospital disinfectants, help fight tuberculosis, and are still effective in the presence of organic materials.
Phenolics act by denaturing proteins and disrupting cell membranes; they also have a bad odor (and can cause skin irritation).
Triclosan is also used in hand sanitizers!
Figure 8.4: Phenols
Dr. Case has a particular distaste for products containing triclosan - while triclosan is not illegal in Singapore, Dr. Case suggests not purchasing triclosan-based products nonetheless!
8.4.2 Alcohols
These are among the most commonly used disinfectants and antiseptics: the most commonly used alcohols are isopropyl alcohol and ethanol.
Alcohols are bacteriocidal and fungicidal (i.e., able to kill fungi), but not sporicidal (i.e., they cannot kill spores).
Alcohols denature proteins and possibly dissolve membrane lipids; they may also be effective against some viruses and are best when used at 70% with water.
Figure 8.5: Ethanol
8.4.3 Iodine (a halogen)
Iodine is a skin antiseptic commonly used in surgery preparations. Iodine oxidizes cell constituents and may kill spores at a high enough concentration.
However, skin damage, irritation, and staining is a problem.
8.4.4 Chlorine (another halogen)
Chlorine oxidizes cell constituents and is used to disinfect swimming pools, in food industries (e.g., dairy products), and are also an effective household disinfectant (e.g., bleach).
Chlorine gas is sporicidal, but it also has the potential to be carcinogenic when combined with other compounds.
8.4.5 Heavy metals
Heavy metals refer to mercury, silver, zinc, arsenic, and copper ions. These metals inactivate and in some cases, precipitate proteins. For this reason, silver coins were used to preserve drinks in the past!
While silver ointment is still used to treat skin infections (and is usually effective), it is usually toxic.
8.4.6 Quaternary ammonium compounds
These are detergents that have antimicrobial activities and are effective disinfectants.
These kill the majority of bacteria by targeting the cell membrane; however, they don’t kill endospores.
Such compounds are inactivated by hard water and soap.
8.4.7 Aldehyde compounds
The most common aldehydes in use are formaldehyde and glutaraldehyde.
Aldehydes are incredibly reactive and are also very toxic. They exhibit antimicrobial activities by inactivating nucleic acids and proteins.
Aldehydes are also sporicidal and can be used as a chemical sterilant.
8.4.8 Sterilizing gases
These gases are used to sterilize heat-sensitive materials and are microbicidal (i.e., kills microorganisms) and sporicidal.
Examples include ethylene oxide sterilization (carried out in an apparatus that looks like an autoclave) and vaporized H2O2 (which combines with proteins and inactivates DNA):
Figure 8.6: Sterilizing Gases
8.5 Mechanical Control of Microbes
8.5.1 Filtration
This reduces the microbe population and sterilizes solutions of heat-sensitive materials by removing microbes.
Examples of this include PM 2.5 filters, liquid filtration in the food industry (e.g., wine, beer, ale, juices, etc.), and research labs.
Membrane filters generally have a pore size of 0.2 microns and are made to filter bacteria. While these filters are used in the food industry and laboratories, such technology is often costly.
HEPA filters (i.e., High-efficiency particulate air) is used in biological safety cabinets and home-filters. Other apparatuses that filter air include surgical masks and cotton plugs on culture vessels.
8.6 Biological Control of Microbes
This an emerging field that has great promise. Some mechanisms include:
- Predation by Bdellivibrio
- Bacteriocins (narrow-spectrum ones, ones that attack membranes, etc).
- Bacteriophages
- Virus-mediated cell lysis involving specific bacteriophage lysins.
8.6.1 Bacteriophages
Bacteriophages express lysins that lyse bacterial peptidoglycan.
Virion-associated peptidoglycan hydrolases make a hole in the cell wall and allow for DNA injection.
Endolysin lyses the peptidoglycan at the end of the cycle to release viruses.
8.6.2 Chemotherapy development
Paul Ehrlich developed the idea of selective toxicity in 1904; he identified dyes that effectively treated African sleeping sickness.
Sahachiro Hata (1910) worked with Ehrlich to identify arsenic compounds that treated syphilis.
Gerhard Domagk, Jacques Trefouel, and Therese Trefouel (1935) discovered sulfa drugs and sulfonamides.
8.6.3 Penicilin
Figure 8.7: Penicilin Mold on a Petri Dish
This is an antibiotic first discovered by Ernest Duchesne in 1896, but his discovery was lost.
Alexander Fleming then accidentally re-discovered it in 1928; he observed peniciling activity on the plate, but he did not think that the drug could be further developed.
In 1939, the effectiveness of penicilin was demonstrated by Florey, Chain, and Heatley. All three scientists then won the Nobel prize in 1945 for the discovery and the production of penicillin.
8.6.4 Chemotherapeutics
Some antibiotics include bactericides, fungicides, algicides, and viricides.
Bacterialcidal agents kill bacteria and many non-pathogens, but not necessarily endospores
Bacteriostatic agents, on the other hand, inhibit the growth of bacteria!
8.6.4.1 General characteristics of antimicrobial drugs
Drugs have the ability to kill or inhibit the pathogen while doing minimal damage to the host. A drug is a narrow-spectrum one if it only works on a few pathogens. On the contrary, a drug is broad-spectrum if it attacks many pathogens at the thereupatic dose.
However, even drugs may have side effects and can become toxic at high-enough concentrations.
The therapeutic index is the ratio of the toxic dose to the therapeutic dose.
The effectiveness of a drug can be expressed in two ways:
- Minimal Inhibitory Concentration (MIC) - the lowest concentration of the drug that inhibits the growth of the pathogen.
- Minimal bactericidal / lethal concentration (MBC or MLC) - this is the lowest concentration of the drug that kills the pathogen.
8.6.4.2 Ability of drugs to reach the site of infection
This depends on the route of administration; drugs can be administered orally, topically (i.e., applied on the skin), intravenously, and intramuscularly.
The ideal drug can be administered orally or intravenously (for rapid action in emergency situations).
A drug can be excluded by blood clots or necrotic (i.e., dead) tissues.
8.7 Determining Antimicrobial Potency
Clincal diagnostics often make use of tests that determine how effective an antimicrobial compound is.
8.7.1 Dilution susceptibility tests
Assuming that nutrient broth is used, tubes of nutrient broth can be spotted on drug-free agar plates to determine cidality (i.e., how well a compound kills microbes).
The MBC is then the broth from which microbes cannot be recovered.
Figure 8.8: A Dilution Susceptibility Test
8.7.2 Disk diffusion tests
Here, disks that are inoculated with specific drugs are placed on agar plates that have the test microbe present.
The drug diffuses into the agar from the disk, establishing a concentration gradient in the process.
The Kirby-Bauer method is the standardized method for carrying out a disk diffusion test; paper disks are used in this test.
Figure 8.9: Disk Diffusion Test
8.8 Mechanisms of Antibiotics
Antibiotics can work in a variety of ways, including but not limited to:
- Inhibiting cell wall synthesis
- Inhibiting protein synthesis
- Inhibiting nucleic acid synthesis
- Inhibiting metabolic pathways
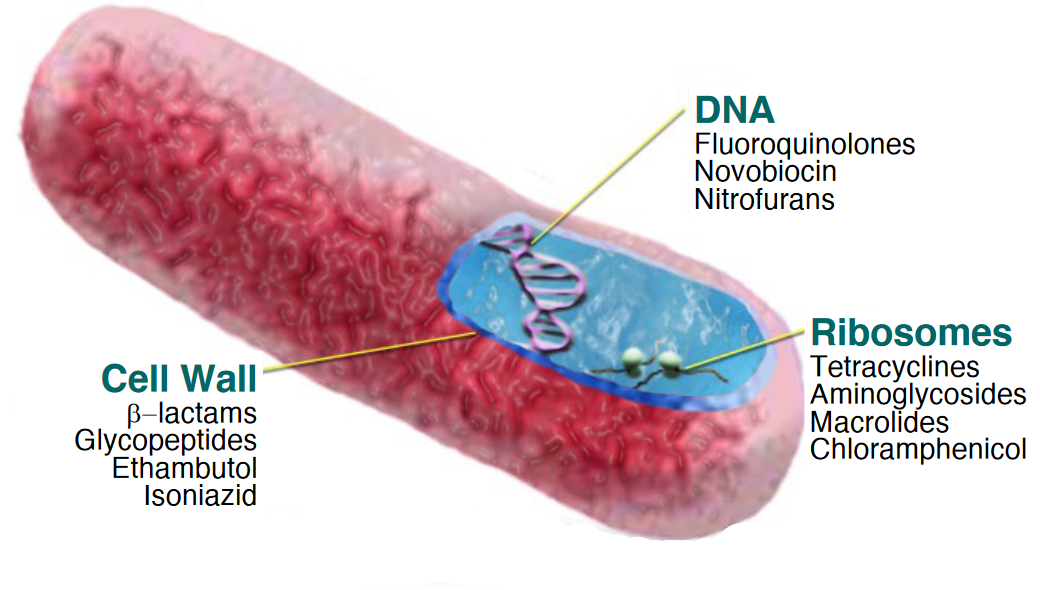
Figure 8.12: Target by Antibiotics
8.8.1 Cell wall synthesis inhibitors
Penicillins (i.e., β lactams) are the most important class of antibiotics for this mode of action - most β lactam antibiotics are 6-aminopenicillanic acid derivatives. Penicillin V and G are narrow-spectrum antibiotics, whereas semi-synthetic penicillin have a broader spectrum than naturally-occuring ones.
Nevertheless, the most crucial feature of such antibiotics are the β lactam ring. Penicillin-resistant organisms produce β-lactamasethat hydrolyzes a bond in this ring:
Figure 8.13: Beta Lactam Antibiotics
Penicillins work by blocking enzymes that catalyze transpeptidation, effectively preventing the synthesis of a complete cell wall. However, penicillins only work on growing bacteria that are synthesizing new peptidoglycan!
It is also worth noting that about 1 to 5% of all adults in the US are allergic to penicillin; allergies to this drug can lead to severe allergic reactions (e.g., anaphylaxis) resulting in death.
8.9 Drug Resistance
This is an ever-increasing problem in today’s world; once originated in a population, it can be transmitted to other bacteria via horizontal gene transfers (e.g., pili conjugation). The resistance to a certain kind of mechanism can also confer resistance to a class of antibiotics.
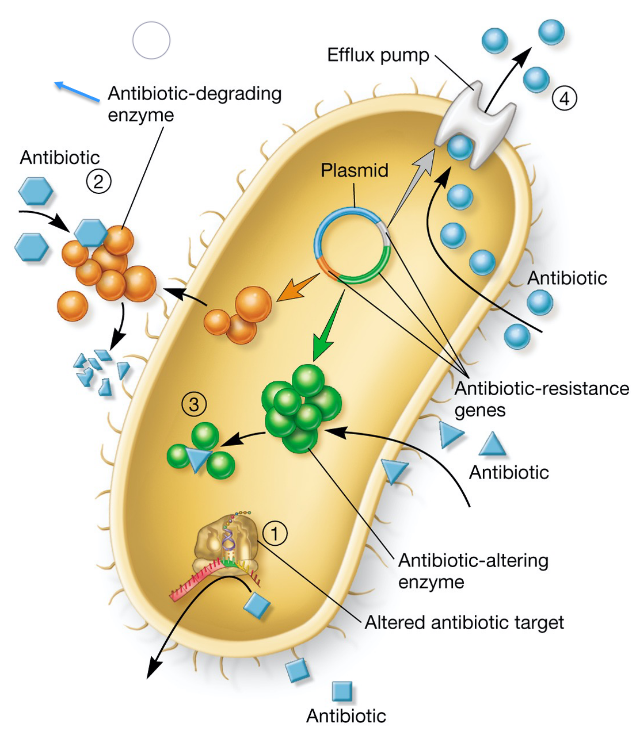
Figure 8.14: Mechanisms of Antibiotic Resistance
As seen above, drug resistance can take on many forms:
- Modification of the target enzyme
- Inactivation of the antibiotic
- Antibiotic-altering enzymes (i.e., the antibiotic is metabolized)
- Drug efflux (i.e., pumping the drug outside of the cell)
- Usage of alternative pathways / enzymes
Mutants that are resistant to antibiotics arise spontaneously; microbes that are present in wounds or abscesses or biofilms may be slow-growing and also resistant to antibiotics.
8.9.1 Origin and transmission of drug resistance
Resistance genes can be found on: 1. Bacterial chromosomes 1. Plasmids 1. Transposons 1. Phages
They can also be freely exchanged between bacteria when found on mobile genetic elements (e.g., DNA).
8.9.1.1 The “superbug”
A methicilin-resistant Staphylococcus aureus (i.e., MRSA) bacterium that develops resistance to vancomycin is a serious threat to human health. MRSA originated from the foot ulcer of a diabetic patient and acquired vancomycin resistance from vancomycin-resistant enterococci.
Vancomycin is a potent last resort antibiotic; vancomycin-resistant S. aureus (i.e., VRSA) are also resistant to most other antibiotics as a result!