Chapter 2 GEOLOGICAL FRAMEWORK
2.1 The southern Appennines
The southern Apennines is a NE-directed fold and thrust belt, with the Bradanic foredeep representing the main depozone of the Pliocene-Quaternary foreland basin system and the Apulian promontory representing the orogenic foreland (Fig. 2.1a-b). According to recent studies (e.g. Mazzoli et al., 2008; and references therein), the Apennine chain consists of two main parts: the Apennine accretionary wedge and the buried Apulian Platform Inversion Belt (APIB). The former, made of sedimentary units detached from their original substrata, includes carbonate platform/slope and pelagic basin successions, stratigraphically covered by Neogene foredeep and wedge-top basin sediments; so, the southern Apennines consist of the superposition of several thrust sheets grouped into three main tectonic complexes (Fig. 3.1a): (1) ophiolite-bearing Ligurian units; (2) Apennine Platform carbonate units; and (3) Lagonegro-Molise deep-marine basin units. The latter form tectonic duplexes and imbricated slices (Fig. 2.1c) overthrusting the buried Apulian Platform carbonates (e.g., Vitale and Ciarcia 2018 and references therein).
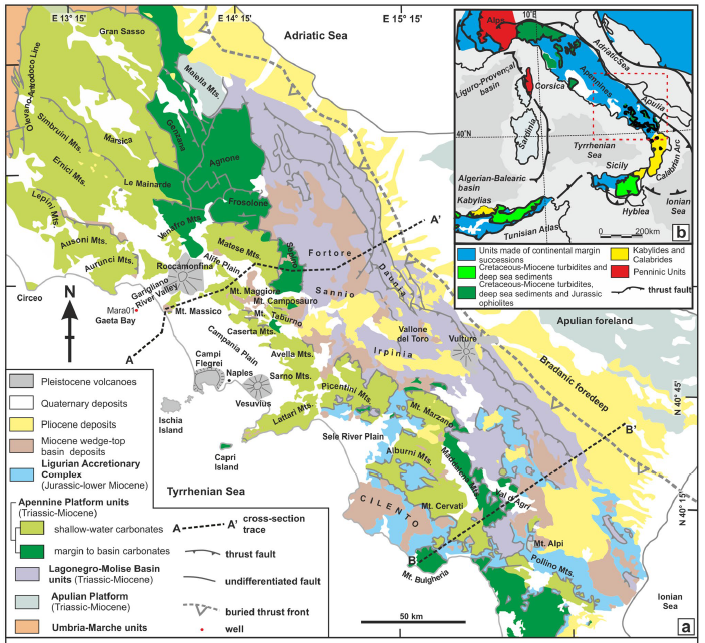
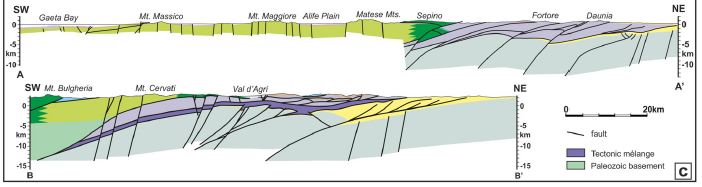
Figure 2.1: A) Geological scheme of the southern Apennines. After Vitale et al. (2018), modified. B) Geological scheme of the Central Mediterranean Sea (after Vitale et al. 2017, modified). C) Geological cross sections. A–A’. after Vitale and Ciarcia (2018) and De Alteriis et al. (2006), modified. B–B’ section after Mazzoli et al. (2008), modified.
Thin-skinned tectonics, characterized by dominant low-angle thrusts, has been active mainly in the first part of the orogenic evolution, from the early Miocene to the early Pliocene, recording most of the orogenic shortening. On the other hand, thick-skinned tectonics, defined by high-angle thrusts, played a significant role in the crustal shortening during the Pliocene–Middle Pleistocene. Thick-skinned tectonics has probably acted through the positive inversion of pre-existing normal faults located in the Permo-Triassic rocks of the downgoing Adria. This orogen is a retreating collisional belt (DeCelles 2012) characterized by a subduction rate exceeding the regional convergence rate with the consequence of a rapid migration of the downgoing slab through the roll-back mechanism (Malinverno and Ryan 1986). Therefore, this chain is the result of the superimposition of the different tectonic units, originating from the Upper Trias in distinct paleogeographic dimensions of the Adriatic passive continental margin and the adjacent Neotethys ocean basin (Fig. 2.2). Normal synsedimentary faults controlled the differentiation of the paleogeographic domains, active during the rifting stages that preceded and accompanied the opening, during Jurassica, of the Neotethid ocean, with the consequent removal of the continental margins Adria (Africa) and Europe
![Schematic stratigraphic logs for the preorogenic and synorogenic successions of the southern Adria domain. The order of logs from left to right approximately reflects the paleogeographic order fromWto E (Figure 2). Labels r efer only to the uppermost Cretaceous–Paleogene rocks. Stratigraphic formations: BS = Breccia a Selce; CBI = Calcari Cristallini; RCG = Roccagloriosa; VLO = Monte Calvello; RDTc = calciruditi a radiolitidi; CT = Colle Trodo; SCZ = Scaglia Detritica; CDZ = Scaglia Cinerea Detritica; SAA = Scaglia Rossa; SCC = Scaglia Cinerea; FMO = Monaco; GOD = Macchiagodena; SGC = Scaglia Condensata; AEN = Calcarenite a Alveoline e Nummuliti; FYR = Flysch Rosso; BL = Lavello; TRN = Trentinara; ALT1 = Calcare del Ciolo; TTG = Calcare di Torre Tiggiano; GSR = Calcari di Castro; PBD = Calcareniti di Porto Badisco; SCA = Scaglia; PES = Peschici; PGB = Brecce di Cagnano [Colour figure can be viewed at wileyonlinelibrary.com]](media/image33.png)
Figure 2.2: Schematic stratigraphic logs for the preorogenic and synorogenic successions of the southern Adria domain. The order of logs from left to right approximately reflects the paleogeographic order fromWto E (Figure 2). Labels r efer only to the uppermost Cretaceous–Paleogene rocks. Stratigraphic formations: BS = Breccia a Selce; CBI = Calcari Cristallini; RCG = Roccagloriosa; VLO = Monte Calvello; RDTc = calciruditi a radiolitidi; CT = Colle Trodo; SCZ = Scaglia Detritica; CDZ = Scaglia Cinerea Detritica; SAA = Scaglia Rossa; SCC = Scaglia Cinerea; FMO = Monaco; GOD = Macchiagodena; SGC = Scaglia Condensata; AEN = Calcarenite a Alveoline e Nummuliti; FYR = Flysch Rosso; BL = Lavello; TRN = Trentinara; ALT1 = Calcare del Ciolo; TTG = Calcare di Torre Tiggiano; GSR = Calcari di Castro; PBD = Calcareniti di Porto Badisco; SCA = Scaglia; PES = Peschici; PGB = Brecce di Cagnano [Colour figure can be viewed at wileyonlinelibrary.com]
Starting from the Eocene, the paleogeographic domains of the southern Appenines have been subjected to compressive deformations referable to the
closure of the Neotethys and the consequent shortening of the passive Adriatic margin. The normal synsedimentary faults have been progressively reactivated and/or truncated by more recent thrust and reverse faults, which have determined the overlapping of the main tectonic units and their transport towards the north-eastern quadrants. The compressive phase lasted troughout the Oligocene-Pleistocene interval, with a progressive migration of the chain front towards the Apulian foreland. Starting from the Upper Miocene, the shortening of the front was accompanied by stretching deformations which truncated and/or reactivated pre-existing thrust and folds associated with them; the development of these structures refers to the opening and evolution of the Southern Tyrrhenian Sea (Doglioni et alii 1996) or to the lifting and consequent collapse of the orogenic wedge (Hippolyte et alii, 1995).
This orogenic chain results from the subduction of the Ligurian oceanic lithosphere beneath the European plate, starting from the Paleocene‐Eocene and the following Miocene‐Middle Pleistocene thrusting and folding affecting the Adria plate. The sinking of the oceanic lithosphere triggered the fast eastward migration of the slab hinge (roll‐back mechanism). In the lower Miocene, an accretionary wedge made up of oceanic deep basin sediments developed (Ligurian Accretionary Complex). During the Miocene, the Ligurian Accretionary Complex overrode the Adria domain forming a new accretionary prism consisting of thrust sheets of the Apennine Platform (basin to shallow‐water) and Lagonegro‐Molise Basin (slope to pelagic) successions. From the Messinian to Middle Pleistocene, the allochthonous pile superposed onto the Apulian Platform domain. During the orogenic crustal shortening stage, from lower Miocene to Middle Pleistocene, the orogenic prism was the locus of clastic sedimentation in several wedge-top basins. The unconformable deposits include the uppermost Burdigalian‐lower Tortonian Cilento Group, the upper Tortonian‐lower Messinian Castelvetere Group (CVTG), the upper Messinianlowermost Zanclean Altavilla Group (AG), the upper Zanclean Baronia Fm and the Piacentian Sferracavallo Fm. At depth, the tectonic pile superposes onto a buried para‐autochthon imbricate belt consisting of the Apulian Platform succession hosting ramp‐dominated thrust faults, which upward crosscut the allochthonous wedge producing out‐of‐sequence structures, frequently deforming the upper Miocene‐lower Pliocene wedge‐top basin deposits (Figg. 2.3-2.4).
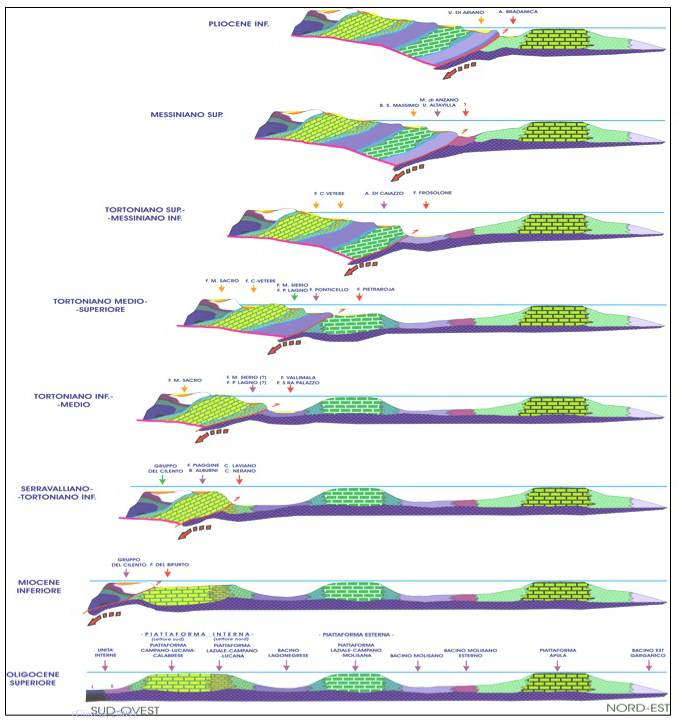
Figure 2.3: paleogeographic reconstruction of Southern Appennines evolution

Figure 2.4: schematic stratigraphic logs of the analyzed units; Vitale et al. 2020
2.2 Geological setting of Monte Maggiore
Monte Maggiore group is part, with Matese complex and Monte Camposauro, of the Upper Triassic–Cenomanian shallow‐water carbonates of the Lazio–Abruzzo platform. This part of the Apennines corresponds to a carbonate platform evolving from the Lias to the Middle Miocene and then transformed by the early Miocene and Pliocene orogeny into the tectonic structures and orographic configuration observed today. It is believed that already in the upper Triassic there was a vast dolomitic platform corresponding to an epicontinental stage, distinguished as a Triassic Apennine platform that between the end of the Triassic and the early Lias, due to a strong rifting phase, was divided into several smaller platforms, while another part of it became the seat of pelagic deposits, evolving into sedimentary basins. Therefore, the resulting platforms corresponded to a second stage defined intraoceanic, with carbonate sediments from infralittoral to supralittoral included in the Lias-Upper Cretaceous-Paleogene interval resumption of carbonate sedimentation in the Miocene and crossed by more depressed areas filled with limestone turbidites. During Dogger and Malm the paleogeographic scheme of the region is the same as during the upper Lias. However, the separation between the Lazio-Abruzzese and Campano-Lucana platforms becomes more evident, infact they were already indicated as the external area and the internal area of the Apennine platform.
The only geological literature relating to the study area is the explanatory notes of the Geological Map of Italy on sheet 172 Caserta (Servizio Geologico d’Italia, 1971; Figg. 2.5-2.6). From a geomorphological point of view, we can distinguish five large areas that correspond to the volcanic building of Roccamonfina, to the lowland of the middle Volturno (Piana di Alife), the Campana Plain, an area of hilly heights that extend to the foot of the southern slopes of the Matese, and lastly, an alignment of Mesozoic limestone-dolomitic mountains, distributed from SE to NW, which includes the mountains of Caserta and the great group of M. Maggiore.
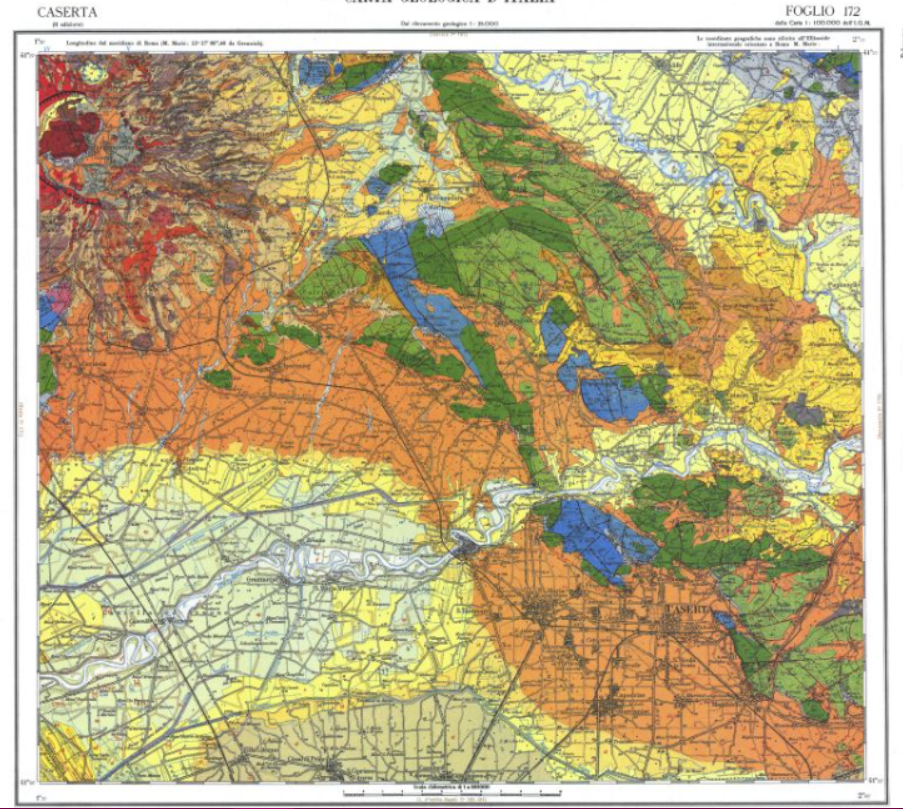
Figure 2.5: Sheet 172 Caserta of Geological map of Italy 1:100.000
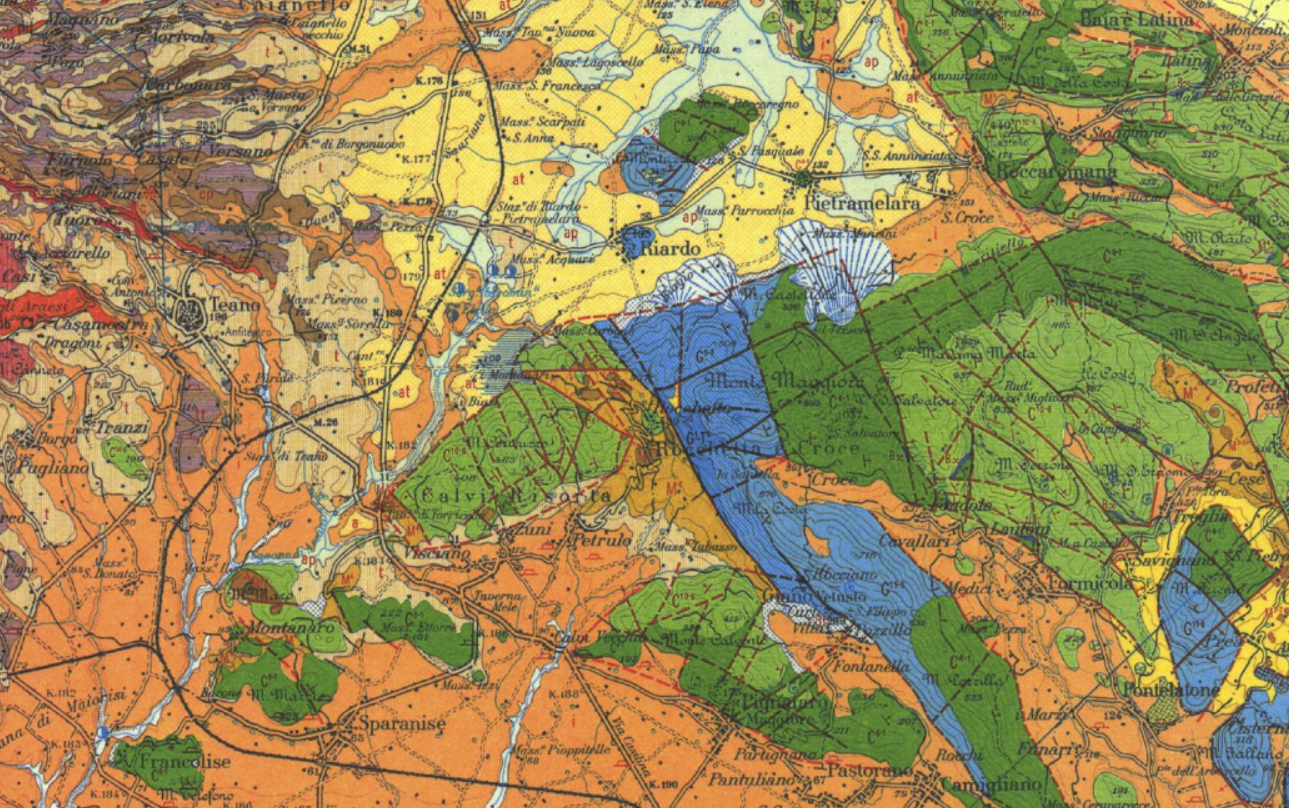
Figure 2.6: excerpt of sheet 172 Caserta of Geologial map of Italy 1:100.000
The lithologies of interest in the study area (Fig.2.6) are:
G1-T5; Dolomite and dolomitic limestone with frequent internal lamination in the upper part (upper Trias): emerges in the basal part of the series and in the western slope of Monte Puritiello (Rocchetta and Croce), where it shows itself with a thickness of about 300 m.
G5-2; Limestones with Paleodasycladus Mediterraneus, brachiopods and spatized fossils. Limestones, conglomeratic limestones, finely detrital limestones, dolomitic limestones and dolomites with Megalodontidae, brachiopods and Orbitopsella in the upper part (Lias inferior-Lias superior): they emerge in regular succession in the Mesozoic series at Monte Puritiello (Rocchetta and Croce) and in the western slope of Monte Costa.
G11-6; Dolomite and detrital dolomitic limestones with gastropods (Lias superior-Malm inferior): a first alternation of dolomitic white or gray dolomitic limestones with oolithic and pisolytic structures alternating with the presence of layers with gastropods and various other microfossils, is found with a thickness of order of 200 m and well exposed in the terminal part of Monte Puritiello (Rocchetta and Croce) and in the basal part of Monte La Costa.
C6-1; White microcrystalline limestones with rare Radiolitidae and sometimes gray flint slates; dark gray conglomeratic limestones (Lower Cretaceous): the succession is discontinuous in the Monte Maggiore group from the Avalanche to the lower Albian, and is then interrupted by a gap corresponding to an emergence phase that lasted until the end of the Cenomanian or at the base of the Turonian ; from the Turonian to the Senonian there is again continuous sedimentation. The gap is marked by a horizon with Bauxite deposits.
Bx; Bauxite horizon and stratiform bauxitic deposits and in lentiform and discontinuous places (Cenomaniano-Albiano / Aptiano): it is found on the last layers of the Requiene level in the Monte Maggiore group and extends from Abruzzo Aquilano to Campania distinguishing the external carbonate platform that it remained emerged during the Middle Cretaceous.
C10-6; Debris limestones with Hippuritidae and Radiolitidae (Upper Cretaceous): the overall thickness is about 200 m and forms most of the reliefs of the M. Maggiore group; in particular it extends into Monte Coricuzzo (Calvi Risorta) and is truncated at the roof by the transgression of the Middle Miocene.
M3-2; Debris limestones from calcarenites to calcirudites mainly organogenic with ostreids, pectinids, bryozoans and lithotamni; breccias and basal conglomerates of the Miocene transgression (Elveziano-Langhiano): this formation is distinguished as limestone from Mastroianni (Tutti-BEN, 1957) and is the equivalent of the Cusano formation; formed by detrital limestone with variable granulometry from area to area. The color is gray or whitish on the exposed surfaces and the clasts are predominantly shells or fragments of various organisms.
M3; Marly limestones and scaly marls with microfauna in Orbulina and Globorotalia in discontinuous and lenticular outcrops (Elveziano): they follow in continuity with the previous formation with the sedimentation of marly limestones and more or less arenaceous marls corresponding to the Longano formation.
M4; Marly and silty clays subtly stratified with alternations of sandstone and pebbly limestone lenses (Tortonian): it is a flyschoid formation that consists of an alternation of clayey-silty marl and sandstone. It corresponds to the formation of Pietraroia and has the same characteristics of the distal phase of the turbid currents (gradation, oblique and convoluted lamination)
i; Ignimbrite of Roccamonfina and Pianura Campana petrographically from trachytic to trachyphonolithic with columnar prismatic fissure (Pleistocene): outside the Campana Plain, the Campanian tuff goes up to surround the eastern foot of the Cretaceous ridge from Baia e Latina to Alvignano and is up to altitudes above 500 m near to Croce (Rocchetta and Croce).
at; Sands and stratified silts, incoherent and humiferous, slow of lapilli and pumice reworked with small dimensions (Holocene): these are very extensive deposits but in slow declivity between the Monte Maggiore and the heights of Pietravairano; furthermore to the south of the sources of Riardo, at the foot of the northern slope of Mount Coricuzzo there is a slab formed by some travertine lenses (tr).
The map (Figg.2.5-2.6) shows that the Mesozoic reliefs are distributed in
an elongated band from SE to NW, in the Apennine direction. The groups of Caserta and Maggiore mountains differ in some stratigraphic details (such as the presence of bauxitic levels that do not interrupt the series in the former) and in some tectonic details that characterize them. In each of the two groups, some subgroups correspond to orographic and structural units (backbone) are different in terms of extension and orientation and separated by some faults, among which, those with more significant morphological influence are directed NW-SE, SW-NE, E-W. The elements of these structures are often hidden by the tuffs resting at the foot of the slopes. In the M. Maggiore group, have been distinguished five ridges and two grabens, corresponding to as many fairly identifiable structural units (D’Argenio and Pescatore, 1963).
To the west is the western Apennine ridge; it extends from Monte la Costa (Rocchetta and Croce) to Monte
Raggeto and consists of the whole succession from the infralias to the
upper Cretaceous. The SW is limited by the large NW-SE
Rocchetta-Bellona fault (object of study of this thesis), whose rejection
increases from SE to NW up to bringing the Infralias dolomites in
contact with the Miocene around Rocchetta. Another NW-SE fault but with
less displacement limits the structure to the NE. The Rocchetta-Bellona
fault divides the first ridge from another subparallel and less evident
because it is fragmented and discontinuous, but always with an Apennine
pattern and corresponds to the roof of the fault itself; this
includes Monte Coricuzzo, Calvento and Tutuli, formed by the lower and
upper Cretaceous. The great eastern ridge extends to the east of the group, also limited by Apennine faults with castings not exceeding 300 m.
Here emerge only Cretaceous limestones, mainly Lower Cretaceous in the northern part, and Upper Cretaceous in the southern one, where there are also extensive transgressive Miocene strips. The ridge includes variously dipping monoclinal blocks due to many E-W or NE-SW faults. Between these two ridges lies the northern ridge, which includes the major hills of the group. Inside it distinguishes a northern part, from M. S. Angelo to P. S. Salvatore (Monte Maggiore), formed mainly by the Lower Cretaceous, and a southern part, from M. Etna to M. Serrone, formed by the upper Cretaceous. A fault with an ENE-WSW direction, with variable and decreasing displacement from W to E, limits this structure towards the Riardo plain. Then we note the central ridge, a subgroup of Mount Maiulo, which includes structures in which the succession of Infralias-Upper Cretaceous outcrops. The structures are limited to the north by reverse faults that overlap the Mesozoic on the Miocene rocks. Finally, the ridge of the Monti di Pietravairano is also ascribed to the Monte Maggiore group, which corresponds to an elongated structure from NE to SW in which successions of different ages emerge, from the Infralias to the Miocene and in stratigraphic and tectonic relationships that are not always recognizable. This ridge is limited by ENE- Tyrrhenian faults; and the fault that limits the ridge to the north, on the north side of Mount Caievola, is an inverse fault that superposes the lower Cretaceous carbonates on the Miocene sandstones and clays. The valley of the S. Giovanni river is defined as the Graben di Formicola, where the tuff cover emerges between the faults that limit the western, northern and central ridges. The flat region between Riardo, Pietramelara and Pietravairano, between the northern, eastern and the Pietravairano mountains is also considered a structural depression, while the heights of S. Felice and the hills of Riardo and Pietramelara would be outcrops of the roof of the lowered plate.
2.3 Fault site
Based on field observations, the section exposed on the site was divided according to the different structural domains that define the internal structure and the fault rock fabric (Caine et al., 1996; Hausegger, 2009). On a genaral general, it is possible to discriminate the following elements:
- Host rock or Protolite: consisting of the mass rock where the original startigraphic succession is preserved.
- Damage Zone: it is a large area characterized by secondary faults with small displacements, veins and sets of shear-fractures, generally related to the growth processes of a fault. Usually, the transition between host rock and damage zone is quite gradual(Fig. 2.7).The bedding and the original structural fabric is commonly preserved and the rock increases its permeability due to the networks of fractures that are created. Characteristics rocks are fault breaches.
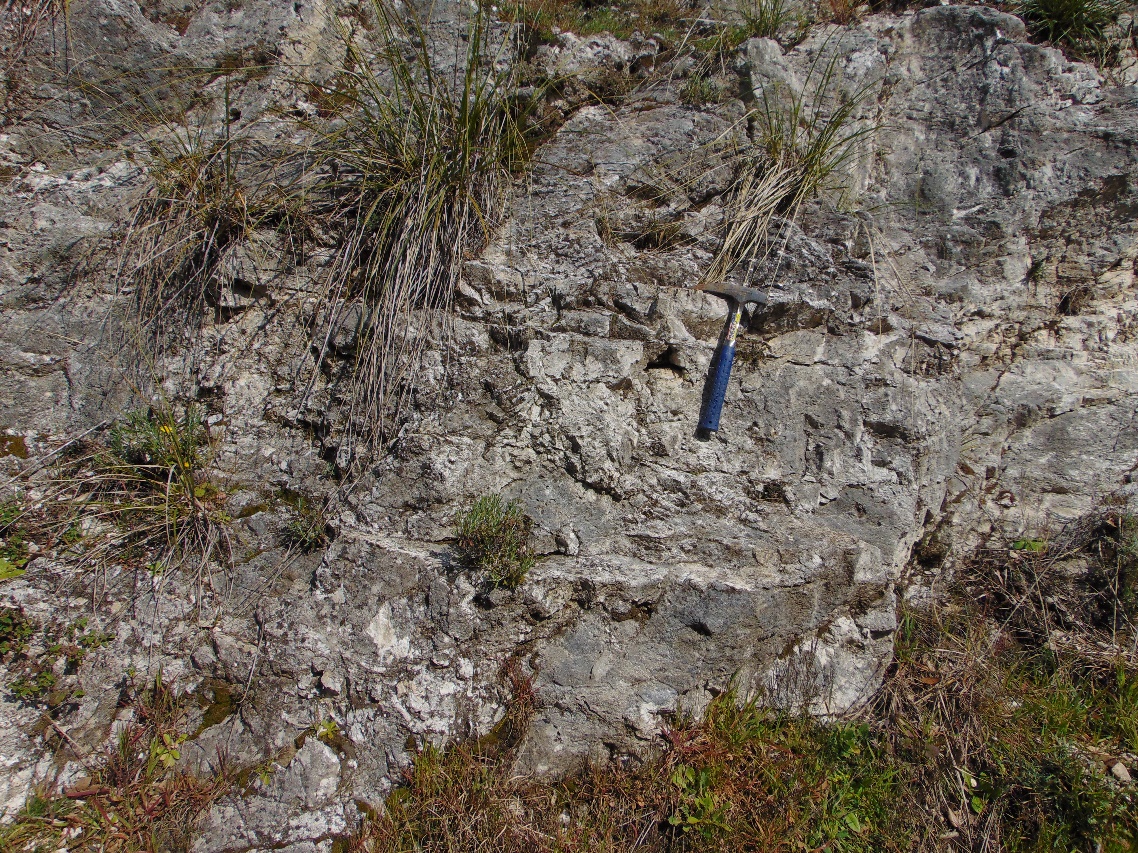
Figure 2.7: Damage Zone
- Fault Core is the area where the maximum displacement of the fault is located, bordering lenticular or tabular volumes of rock around slip surfaces (Sibson, 2003). Its interior is characterized by the maximum degree of fracturing (Fig. 2.8), by the presence of phenomena of rotation of the clasts and from the comminution of grain that comes to obliterate the original fabric of the cashier. The concentration of the slip localization in fault core creates volumes with low permeability. The rocks present in the core are called Cataclasites or Ultracataclasites depending on the grade of comminution.
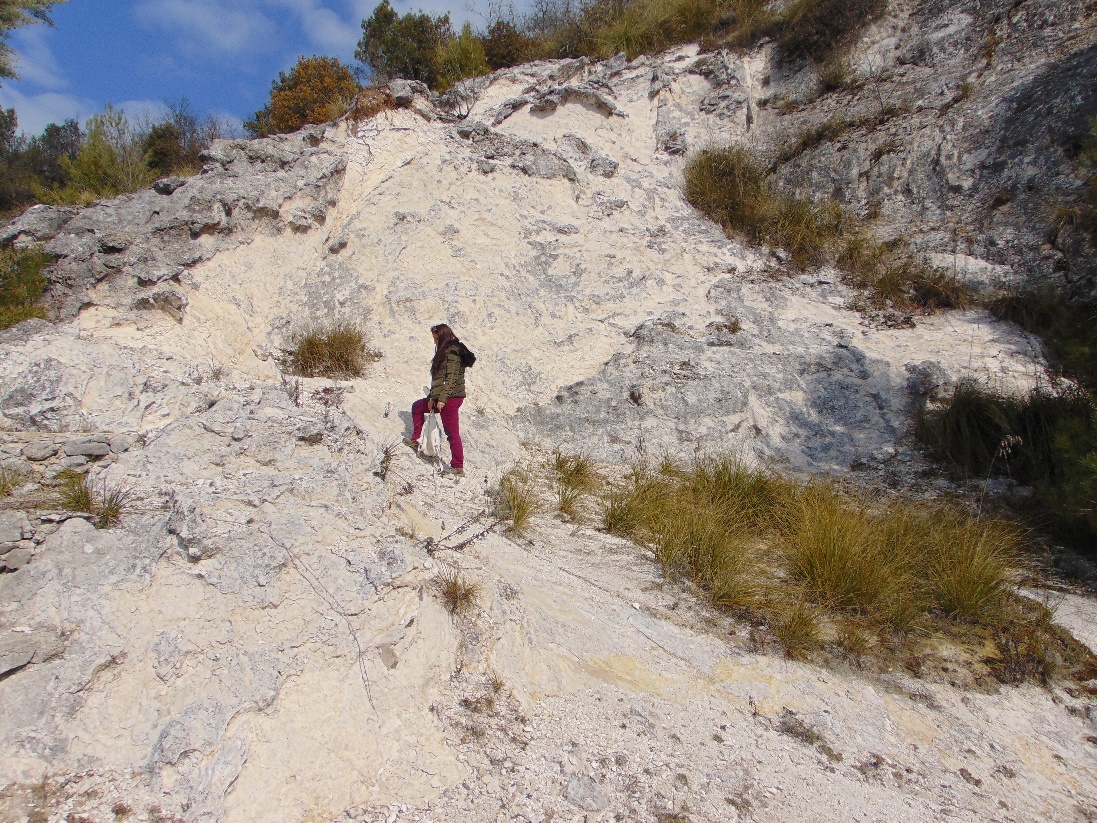
Figure 2.8: Fault core transept
Since the fault core develops within the damage zone, zoning space (from protolite to core) corresponds to different evolutionary steps of propagation of a fault. When faults develop within sedimentary carbonate sequences, their structure is strongly controlled by the presence of inherited anisotropies such as layer joints, veins, joints and pressure-dissolution surfaces (Graham et al., 2003; Agosta and Aydin, 2006; Kurz et al., 2008; Hausegger et al., 2010). The evolution of the fault zone involves the progressive interaction of these inherited structures, leading them to slide over each other and develop new slip surfaces and zones.